Quantum Leap: How Quantum Computing is Poised to Revolutionize the Tech Industry
- Martin Low
- 5 days ago
- 20 min read
Quantum computing is an emerging field that harnesses the strange rules of quantum physics to process information in fundamentally new ways. Unlike today’s classical bits (which are either 0 or 1), quantum bits or qubits can exist in superpositions of 0 and 1, enabling many calculations to be carried out simultaneously. In addition, qubits can become entangled, meaning they share information instantaneously across distance. These effects allow quantum computers to tackle certain problems far beyond the reach of even the fastest conventional supercomputers. Although still in their infancy, quantum computers promise transformative benefits in encryption, chemistry, optimization, and more. In this paper, we dive deep into how quantum computing works, explore the leading technologies and companies, and examine the practical applications that could revolutionize industries.
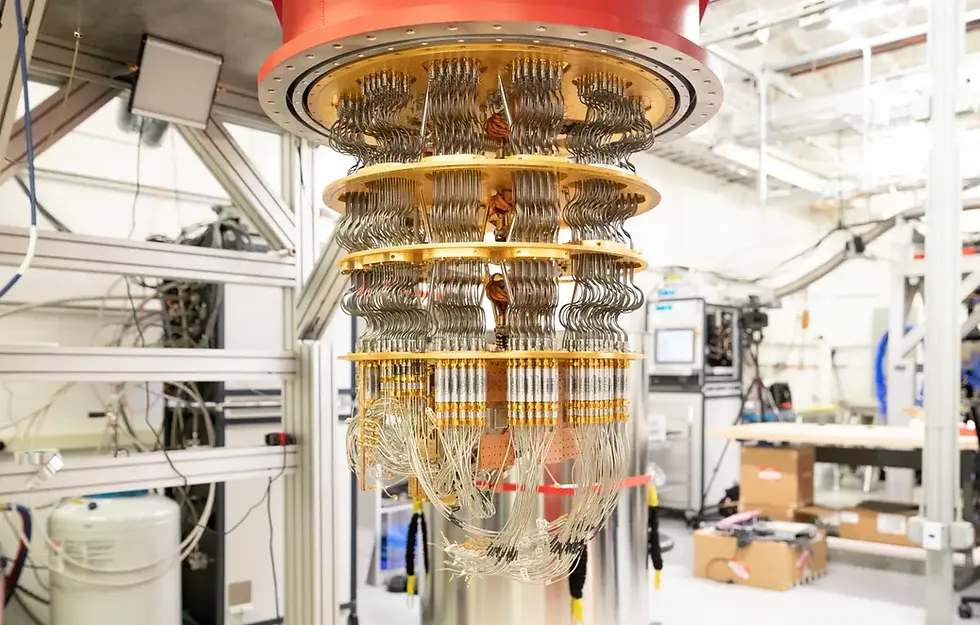
Understanding Quantum Computing
To understand quantum computing, it helps to first know what a qubit is. A qubit is the quantum analogue of a classical bit, but it can represent 0, 1, or any quantum superposition of those states. You can think of a qubit like a spinning coin: while spinning it is both heads and tails in a way, collapsing to one or the other when observed. Physicists typically implement qubits using tiny physical systems such as individual atoms, ions, photons of light, or circuits made of superconducting metal. For example, IBM explains that “superconducting qubits” are circuits cooled to millikelvin temperatures, valued for their speed and precise control. Alternatively, trapped ions – charged atoms suspended in vacuum by electromagnetic fields – act as qubits with exceptionally long coherence times.
Building a working quantum computer is extremely challenging. Qubits are very sensitive: interactions with the outside world can easily disrupt their quantum state (a problem called decoherence). For this reason, many quantum processors must be kept at ultra-cold temperatures (often just a few thousandths of a degree above absolute zero) in specialized refrigerators. Others rely on photons, which can operate at room temperature and travel over fiber. Crucially, quantum computers must be carefully isolated and controlled by microwave pulses or lasers to perform computations.
Quantum algorithms work very differently from classical ones. In essence, they prepare qubits in superposition, apply a sequence of quantum gates (operations) that entangle and interfere the qubit states, and then measure the result, collapsing the system to a classical output. This process can yield answers much faster for some hard problems. For example, Shor’s algorithm (a quantum algorithm) can factor very large numbers exponentially faster than the best known classical algorithms, threatening current encryption schemes. Or Grover’s algorithm can search an unordered database quadratically faster than classical search. While we will not delve into these math details here, the bottom line is that for certain tasks – like breaking RSA encryption or simulating a molecule’s energy – a sufficiently powerful quantum computer could drastically outperform classical machines.
In the coming sections, we will survey the major types of quantum hardware and companies (each of which uses different qubit technology), compare their capabilities, and illustrate practical uses in areas such as cryptography and drug discovery. We will also include expert perspectives and examples to make these ideas concrete.
Quantum Technologies: A Deep Dive
Scientists and engineers around the world are building quantum processors using several different hardware approaches. Each approach uses different physical qubits and has its own advantages and challenges. The main ones are superconducting circuits, trapped ions, photonic systems, and topological qubits. We describe each type below.
Superconducting Quantum Qubits
Most of the current quantum hardware from companies like IBM, Google, and Rigetti is based on superconducting circuits. These devices are essentially tiny electrical circuits made from superconducting metals (like aluminum) that are cooled down to near absolute zero. At those temperatures, electrons pair up and flow without resistance, allowing quantum effects to dominate. In practice, a superconducting qubit is implemented by a loop of superconducting wire interrupted by Josephson junctions (tiny insulating gaps). The current and voltage in this loop can be in a superposition of 0 and 1 states.
Superconducting qubits are favored for their fast gate speeds and relatively straightforward fabrication (they can be manufactured using standard chip-making techniques). As IBM explains, “superconducting qubits… operate at extremely low temperatures” and are “favored for their speed in performing computations and fine-tuned control”. However, they suffer from shorter coherence times (they lose their quantum state in microseconds unless carefully isolated) and require bulky dilution refrigerators to stay cold.
A silicon wafer etched with an array of superconducting quantum processor chips (image: Steve Jurvetson/CC BY 2.0). Many quantum processors (for example those made by D-Wave and others) are fabricated like conventional microchips. This wafer contains many copies of a superconducting qubit chip, each featuring tiny square circuit elements. These chips are operated at millikelvin temperatures inside large cryogenic refrigerators.
In a typical superconducting quantum computer, each qubit is controlled by microwave pulses and is connected by on-chip wiring to its neighbors. The state of the qubits can be changed and entangled by applying carefully timed microwave signals. After a series of gates (the basic operations of the quantum algorithm), the qubits are measured by coupling them to resonators or detectors. The readout gives a classical result (0 or 1) for each qubit, but the strength of quantum algorithms is that they have explored many possibilities in superposition before this final measurement.
A real-world example is IBM’s Quantum System One, which contains multiple superconducting qubit chips in a large refrigerator. Such systems are housed in special research labs. For instance, IBM’s premiere research lab at Yorktown Heights (the Thomas J. Watson Research Center) contains multiple IBM Quantum systems and an experimental supercomputer. This photo shows one of those refrigerators in the Yorktown Think Lab:
A dilution refrigerator for an IBM quantum computer at the IBM Yorktown Heights lab. Each dangling metal plate contains superconducting quantum circuits (qubits). IBM reports that its labs “house multiple IBM Quantum systems”, which are examples of the superconducting qubit approach. The gold-colored plates are stages at progressively lower temperatures; the bottom plate (not visible here) reaches roughly 15 millikelvin, near absolute zero.
Superconducting qubit systems have achieved the highest qubit counts to date in gate-model devices (e.g. Google’s 54-qubit “Sycamore” processor, IBM’s 65+ qubit machines, etc.). For example, IBM recently unveiled a 100+ qubit system (their “Osprey” chip, 127 qubits) and plans to scale further. (Though qubit count is not the only metric, it gives a sense of progress.) Companies like Rigetti have built systems on the order of dozens of qubits, and are working toward hundreds.
In summary, superconducting quantum processors use well-understood chip technology but require extreme cooling. They are currently the most mature approach in terms of qubit count and control, but scaling them to millions of qubits (and sufficiently long coherence for complex tasks) remains a challenge of engineering and materials.
Trapped-Ion Quantum Systems
Another leading approach is trapped-ion quantum computing, pioneered by companies like IonQ and Honeywell. In this approach, each qubit is a single electrically charged atom (ion) floating in free space. Using electromagnetic traps (tiny electrode arrangements), researchers hold a line of ions stationary within a vacuum chamber. Lasers are then used to manipulate the quantum states of these ions and entangle them.
Trapped ions have some striking advantages. Each ion is nearly identical and well-isolated, which means qubit-to-qubit variability is very small. Importantly, trapped-ion qubits can have extremely long coherence times (seconds or more in some cases), far longer than in superconducting circuits. This makes them very precise and robust, although they tend to operate more slowly (gates can take microseconds to milliseconds).
IBM’s quantum computing primer notes that “trapped ion particles… are noted for long coherence times and high-fidelity measurements”. In practice, companies like IonQ trap a few dozen Ytterbium or Calcium ions in a vacuum chamber. They then shine laser beams on the ions: these lasers flip the state of each ion (performing qubit gates) or create entanglement through shared vibrations of the ion chain.
A cryogenic trap and optical equipment for a trapped-ion quantum computer (photo: Nilhope/CC BY-SA 4.0). This image shows the golden structure of an IonQ (Alice & Bob) trapped-ion quantum computer. Each suspended level contains electrodes and wiring to trap the ions, and lasers (not pictured) shine on the ions from the sides. As one Wikimedia description notes, this is “a quantum computer cryostat in the Lab at Alice & Bob HQ”. While it looks similar to superconducting fridges, the key difference is that the qubits inside are ions held by electric fields, not superconducting circuits.
In trapped-ion machines, all-to-all connectivity is possible (each ion can be entangled with any other ion via collective modes), which is a plus for many algorithms. However, scaling up to large numbers of ions is challenging because the trap size and laser requirements grow, and operations become slower. Currently, IonQ’s flagship devices are on the order of 25 to 36 qubits (called “Aria” and “Forte”), each of which are available through cloud platforms. Despite fewer qubits than some competitors, the high fidelity and long memory make them powerful for precision tasks.
Photonic Quantum Computers
A very different approach is to use photons – particles of light – as qubits. Photonic quantum computing has a natural advantage of room-temperature operation and easy connectivity (photons travel through optical fibers). Companies like Xanadu are leading this field, using laser light, optical fibers, and detectors. In a photonic quantum computer, qubits can be encoded in properties of photons (like the number of photons, their phase, or polarization). A sequence of beam splitters, phase shifters, and squeezers (nonlinear crystals) acts as the quantum gates.
Photonic systems often use a specific task called boson sampling or Gaussian boson sampling to show quantum advantage, because general photonic gate-model computers are still in development. Recently, Xanadu’s Borealis photonic processor (with 216 squeezed-light modes) performed Gaussian boson sampling on a scale far beyond classical reach. As reported in Nature, Borealis required only 36 microseconds to produce a sample that would have taken an estimated 9,000 years for the best classical supercomputers – over 50 million times faster.
Photonic qubits have the appealing property that they can operate at or near room temperature (no dilution fridge needed). IEEE Spectrum notes that photonic quantum computers can, “in principle, operate at room temperature,” and can be integrated into fiber-optic telecom infrastructure. They are seen as a promising path toward scaling to very large numbers of qubits, since light beams can be manipulated precisely and multiplexed in time and space. On the flip side, single-photon sources and detectors must be highly efficient, and error correction is still an active research area.
Xanadu has made photonic quantum computers available in the cloud. In 2020, they announced the first public photonic quantum cloud: “Applicants can access 8, 12, and soon 24 qubit machines over the cloud,” according to IEEE Spectrum. They used this platform to demonstrate boson-sampling advantage. Beyond that, Xanadu unveiled Aurora, a prototype for a scalable photonic quantum computer with a modular architecture (hundreds of photonic chips networked together).
In summary, photonic quantum computing uses lasers and optics instead of matter qubits. This approach is well-suited for quantum communications (quantum cryptography already uses photon-based QKD over fiber) and shows promise for computation with the potential for massive parallelism. As Xanadu’s work shows, it is now possible to achieve quantum advantages with light, validating photonics as a future platform.
Topological Quantum Computing
A more speculative but highly intriguing approach is topological quantum computing. This method aims to make qubits that are intrinsically protected from errors by the geometry (topology) of their quantum state. The idea goes back to proposals for Majorana zero modes and anyons in certain exotic materials. Microsoft, in partnership with groups at UC Santa Barbara and other labs, has been a leader in this approach.
In topological quantum computing, a qubit would be stored nonlocally in a pair of “Majorana” particles at the ends of a superconducting wire or in defects of two-dimensional materials. Because the information is stored in a global property of the system, it is naturally immune to many local disturbances. This promises more robust qubits that need much less error correction overhead. For many years, the field was theoretical, but there have been exciting experiments.
In early 2025, Microsoft announced Majorana 1, claimed as “the world’s first quantum processor powered by topological qubits”. This device uses a special material (“topoconductor”) to host Majorana modes. Microsoft says Majorana 1 is designed to scale to “a million qubits on a single chip” (in principle). They also reported experimental evidence of “hardware-protected topological qubits” (meaning the qubit was smaller, faster, and showed digital control). This is very early stage, but it is a major milestone: topological qubits could greatly reduce the error rate problem if they can be scaled.
Topological quantum computers would still run quantum gates (via braiding Majoranas, for instance), but those gates could be much more fault-tolerant. We have not yet seen a full-scale topological quantum computer, but the field is advancing. In summary, topological approaches aim to solve the key scaling problem (error correction) from the physics side. They remain experimental, but they highlight the creativity in quantum hardware research.
Quantum Computing Platforms and Companies
Many companies and research groups are developing quantum hardware, software, and cloud platforms. Some of the most notable players today (beyond Google and Microsoft research) include IBM, Rigetti, IonQ, D-Wave, and Xanadu. Each has its own approach, and all provide some form of cloud access to users or partners. We briefly review these platforms, especially noting how students and researchers can try them out.
IBM Quantum
IBM has been a pioneer in quantum computing, starting with the IBM Quantum Experience in 2016 (one of the first cloud quantum platforms). IBM’s current hardware uses superconducting qubits. As of 2025, IBM’s portfolio includes chips like Osprey (127 qubits) and a roadmap up to 1000 qubits in coming years. They have built a global cloud called IBM Quantum® with a “100+ qubit quantum processing units” offering. Importantly, IBM provides easy access: one can sign up on the IBM Quantum Platform and get free access (10 minutes per month) to their quantum computers. This is ideal for students and educators. The platform uses Qiskit (an open-source SDK) to program the devices. IBM also offers extensive tutorials, textbooks, and even full courses on Qiskit. In short, IBM’s ecosystem is very student-friendly: free tier access, documentation, and a large user community.
For example, IBM’s quantum cloud page advertises: “Sign up today to get 10 free minutes of execution time per month on our 100+ qubit [systems]”. In addition to free access, IBM provides paid plans for more project time, consulting, and integration. It also emphasizes quantum-safe cryptography tools through its IBM Quantum Safe initiative. In summary, IBM Quantum is a leader with mature hardware and a well-established cloud platform. (IBM also collaborates with educational programs: for instance, it offers a Qiskit Textbook and quantum courses on edX.)
Rigetti Computing
Rigetti Computing is a startup focused on superconducting qubit processors, founded in 2013. They developed the Forest platform early on and now work with cloud providers. Rigetti’s chips (named Aspen) have on the order of tens of qubits (e.g. an 80-qubit design) and they plan to scale further. Rigetti offers access to its quantum processors via AWS Braket and Microsoft Azure Quantum.
Notably, Rigetti and Microsoft Azure offer promotional credits. Rigetti’s website mentions “take advantage of $500 in free credit for Rigetti hardware” on Azure Quantum. This means students and researchers can apply the free credits to run code on Rigetti’s QPUs. They also mention a $10,000 grant for larger projects and free access via Strangeworks QC (a collaborative quantum cloud). In summary, Rigetti’s approach includes open source tools (like pyQuil) and accessible cloud access with initial free credits.
IonQ
IonQ (which was originally funded by DARPA and others) makes trapped-ion quantum computers. IonQ’s systems, like Aria and Forte, have on the order of 25 and 36 qubits respectively. They focus on delivering high-fidelity quantum hardware via cloud services. IonQ’s devices can be accessed through various cloud marketplaces: AWS Braket, Microsoft Azure, and Google Cloud.
For students, IonQ also provides free tiers and academic programs. For early exploration, IonQ allows sign-ups for free simulator access and basic testing. For heavier use, IonQ’s website notes that researchers can apply for up to $10,000 in research credits. (This is similar to Rigetti’s Azure credit, encouraging academics to try out their hardware.) IonQ also joined the IBM Quantum Network as a member, indicating collaborations. In practical terms, a student could use Google’s Cirq or AWS Braket to run jobs on IonQ’s devices, with small fees per-shot. IonQ also publishes pricing (e.g. on AWS) showing per-task and per-shot costs, but the key point is that small-scale access is readily available.
D-Wave and Quantum Annealing
D-Wave is a Canadian company that has been selling quantum annealers since 2011. Unlike the gate-model devices above, D-Wave’s machines use quantum annealing (a type of adiabatic quantum computing) aimed at optimization problems. D-Wave’s latest Advantage system contains on the order of 5760 qubits arranged in a “Pegasus” connectivity graph. (These are superconducting flux qubits at 15 mK, but they function differently than gate qubits.) The key is that D-Wave’s machines can handle much larger qubit counts today, but they solve only specific problems (finding ground states of Ising spin models), rather than performing arbitrary quantum circuits.
D-Wave offers cloud access through its Leap platform. Anyone can sign up for a free Leap account, which provides a trial plan allowing users to run demos on the quantum annealer. Educational and research users can apply to the Leap Quantum LaunchPad program for extended access (with educator grants, etc.). In short, D-Wave is unusual because it has the largest machines and a widely open cloud program (Leap) that even has forums and community resources. Pricing is based on solve time or leasing nodes; D-Wave’s own materials note that Leap free accounts can run pre-built examples to learn the system.
While D-Wave’s technology is not directly solving cryptography or simulating molecules, it is already used in fields like logistics optimization, machine learning (quantum annealing algorithms for clustering), and materials science. The fact that they offer thousands of qubits means a student or company can experiment at a scale impossible with other platforms today. We will see later that even an annealer could potentially speed up certain algorithms related to daily life (like scheduling or network design).
Xanadu Quantum Computers
Xanadu is a startup based in Toronto that builds photonic quantum hardware. As discussed above, they use beams of light in optical fiber and nonlinear crystals to create and manipulate quantum states. Xanadu’s major products are accessible via the cloud. In September 2020, Xanadu released the world’s first public photonic quantum computing platform, as reported by IEEE Spectrum. At that time, users could apply to use 8-qubit or 12-qubit photonic machines (with a 24-qubit system coming soon).
Xanadu provides cloud access through their own Quantum Cloud, and they also had their Borealis processor (216 modes) available on AWS Braket (though it was later retired on Braket in 2023). They emphasize that their photonic QPUs operate at room temperature and could scale to very large sizes. Xanadu also offers an open-source quantum software library called PennyLane, which is popular for quantum machine learning. While Xanadu’s current accessible devices have modest qubit counts, they demonstrate cutting-edge photonic capabilities and support student learning through their cloud and docs.
In addition to these five, other players include Google, Microsoft (Azure Quantum), Amazon (Braket), and various startups (e.g. PsiQuantum, Pasqal with neutral atoms). Google, for example, uses superconducting qubits (the Sycamore chip) and recently announced a new 60-qubit cold qubit "Borealis" chip (different from Xanadu's) for experiments, but Google’s devices are not generally open to public outside research collaborations. Microsoft is more focused on topological qubits internally but provides a cloud interface (Azure Quantum) to other providers (Rigetti, IonQ, Quantinuum, etc.). Amazon Braket is a marketplace that lets users access many of the above QPUs and simulators (IBM, Rigetti, IonQ, D-Wave, Xanadu, and even startups like QuEra) through one portal, usually with a pay-per-use model.
In the table below, we summarize these leading platforms:
Platform / Company | Qubit Technology | Qubit Count (device) | Access / Pricing | Notes and Features |
IBM Quantum (IBM) | Superconducting circuits | 100+ (e.g. 127) | Cloud (free 10 min/month on 100+ qubit QPUs) | Free tier via IBM Quantum Platform; uses Qiskit; large user community. |
Rigetti Quantum | Superconducting circuits | ~80 (Aspen) | Cloud via AWS/Azure (free $500 credit on Azure) | Open Forest/pyQuil SDK; $500 free credit, $10k academic grant. |
IonQ | Trapped-ion (Yb ions) | 25–36 (Aria, Forte) | Cloud via AWS Braket/Azure Quantum (free simulator; research credits) | Trapped ions -> high fidelity; up to $10k research credits. |
D-Wave (Leap) | Quantum annealer (flux qubits) | 5760 (Advantage) | Cloud via Leap (free trial plan) | Optimization problems; thousands of qubits; free demos & LaunchPad. |
Xanadu Cloud | Photonic (squeezed light) | 8, 12 (24 soon) | Cloud (public access; pay-as-you-go) | Room-temp operation; first photonic QC cloud; PennyLane software. |
Table: Comparison of leading quantum computing platforms. Qubit counts are approximate for current devices. Access/pricing notes include free trial or credits where available.
Each company’s platform comes with its own ecosystem: IBM Qiskit and tutorials; Rigetti’s Forest/pyQuil; IonQ’s Cirq and high-level SDKs; D-Wave’s Ocean tools for annealing; Xanadu’s PennyLane and Strawberry Fields. Many of these have free educational tiers to help students and researchers start exploring quantum algorithms on real hardware.
Practical Applications of Quantum Computing
Quantum computers are not meant to replace classical computers for everyday tasks; instead, they aim to tackle specific problems that are intractable classically. Here are some of the most-discussed application areas:
Cryptography and Security: One of the earliest known quantum algorithms was Shor’s algorithm for factoring large numbers. Since many encryption schemes (like RSA) rely on factoring, a large-scale quantum computer could break current encryption. NIST explains that while a classical computer might need “billions of years” to factor a 2048-bit RSA key, a sufficiently powerful quantum computer could do it in days or even hours. This prospect has spurred a worldwide push for post-quantum cryptography (new algorithms secure against quantum attacks). However, quantum also offers new security methods: for example, Quantum Key Distribution (QKD) uses the properties of photons to create provably secure communication links. In practice, while general-purpose quantum computers still need millions of qubits to threaten real cryptosystems, the field of quantum-resistant algorithms is already advancing in anticipation.
Drug Discovery and Pharmaceuticals: The pharmaceutical industry spends billions on R&D to design new drugs, often using complex simulations of molecular chemistry. As McKinsey reports, drug discovery is a natural fit for quantum computing because “the molecules… are actually quantum systems” and quantum computers can simulate their atomic interactions accurately. In theory, a quantum computer could model the exact energy levels and reaction dynamics of a candidate drug molecule far more precisely than classical computers can. This could drastically speed up the identification of promising compounds. Researchers have already begun partnering with quantum companies for this purpose. For example, Xanadu’s photonic computers and IBM’s QPUs have been used in pilot projects to simulate simplified molecules (like hydrogen chains) and optimize protein folding metrics. Though we are not yet at the point of designing an approved drug on a quantum computer, the potential is huge: McKinsey calls it “game-changing” for pharma to simulate larger, more complex molecules with QC.
Materials Science and Chemistry: Similar to drugs, designing new materials (for batteries, catalysts, superconductors, etc.) requires understanding quantum behavior at the atomic level. Quantum computers could enable simulation of exotic materials and discovery of new compounds for energy storage or electronics. For instance, quantum algorithms might help find better battery materials by simulating electrode-electrolyte interactions. This overlaps with the pharmaceutical use case but is relevant to industries like automotive and renewable energy. Indeed, experts note that problems in material chemistry and biology are especially well-suited for quantum advantage.
Optimization and Machine Learning: Many real-world problems can be phrased as optimization tasks or probabilistic sampling. Examples include routing trucks, scheduling flights, optimizing financial portfolios, or training certain types of machine learning models. Quantum annealers (like D-Wave) and gate-model devices can both tackle small optimization problems (like solving Quadratic Unconstrained Binary Optimization, QUBO, forms). Tech companies explore quantum-enhanced AI – for instance, using quantum circuits to speed up parts of training or sampling in artificial intelligence. As Alice & Bob cofounder Théau Peronnin notes, industries like finance have long used supercomputers to push the frontier, but sectors like pharmaceuticals and batteries could be transformed by the new scale that quantum offers. Quantum machine learning is still nascent, but some hybrid quantum-classical algorithms (like QAOA for optimization, or using quantum circuits in neural networks) are being tested on current hardware.
Education and Research: Finally, we should not overlook the educational impact of quantum computing itself. Universities and online platforms are increasingly offering courses in quantum information science. For instance, students can now take MOOCs on Coursera, edX, or Khan Academy about quantum computing, often using IBM’s or Microsoft’s learning materials. Many undergraduate physics and engineering programs have started quantum labs where students program actual quantum processors. Companies support this by providing free or low-cost access: IBM, Rigetti, IonQ, and D-Wave all have outreach programs and documentation aimed at students. The Quantum Education Hub (a CERN collaboration) and similar initiatives curate learning materials. In short, quantum computing has become a subject taught at top universities and even high schools, preparing the next generation of scientists and engineers.
The key point is that current quantum computers (often called NISQ – Noisy Intermediate-Scale Quantum – devices) are still error-prone and small, so practical breakthroughs are limited right now. However, they offer valuable testing grounds. Students and researchers can experiment with real hardware to develop quantum algorithms. Moreover, companies are already partnering with scientific labs: for example, Volkswagen used D-Wave’s annealer for traffic flow problems, and biopharma firms have run pilot programs on IBM and IonQ QPUs for molecule optimization. These serve as important proofs-of-concept.
Expert insight: In a recent interview, quantum computing entrepreneur Théau Peronnin emphasized that “quantum will always be paired with classical computing” but that quantum offers “new breadth” in computing power. He notes that some industries (finance, AI infrastructure) are already pushing classical resources, but fields like pharma and batteries could be completely transformed once quantum scales. This reflects a common view: quantum will augment existing computing, not replace it, and will unlock improvements in fields that involve chemistry and complex optimization.
Comparison of Quantum Computing Platforms
To summarize and compare the major platforms discussed above, the following table lists their key properties:
Platform/Provider | Technology | Qubits (scale) | Access/Pricing | Notes |
IBM Quantum | Superconducting circuits | 100+ (e.g. 127) | Cloud (free plan: 10 min/month on ≥100-qubit QPUs) | Extensive software (Qiskit); IBM Q Network; education focus. |
Rigetti Computing | Superconducting circuits | ~80 (Aspen series) | Cloud via AWS/Azure; $500 free credit (Azure Quantum) | PyQuil/Forest platform; plans for larger chips. |
IonQ | Trapped Yb ions | 25–36 (Aria & Forte QPUs) | Cloud via Braket/Azure/Google; free sim + research credits | High fidelity, long coherence; members of IBM Q Network. |
D-Wave (Leap) | Quantum annealer (flux qubits) | 5760 (Advantage) | Leap cloud (free trial plan); paid plans; Hybrid API. | Special-purpose optimization machine; thousands of qubits. |
Xanadu Quantum Cloud | Photonic (squeezed light modes) | 8, 12 (24 coming) | Public cloud (free signup, pay per use) | Room-temp operation; first photonic cloud; PennyLane SDK. |
Table: Leading quantum computing platforms and their characteristics. Qubit counts are illustrative. “Access/Pricing” highlights free/education options where applicable.
Aside from these, major tech companies have their own programs (Google Quantum with 54+ qubits for collaborators, Microsoft Azure Quantum bridging to multiple vendors, Amazon Braket marketplace). The ecosystem is rapidly evolving, but the table above captures the current standouts.
How Quantum Computing Impacts Daily Life and Industries
What does all this mean for individuals and society? We are still at the dawn of the quantum era, but the potential impact is enormous:
Daily technology: In the immediate term, most consumers won’t notice a “quantum chip” in their phone or laptop anytime soon. However, there could be indirect effects. For example, once quantum computers become powerful enough to break conventional encryption, we will transition to quantum-resistant cryptography (affecting internet security, banking, etc.). Conversely, quantum key distribution (QKD) could secure critical infrastructure communications (like government or financial networks). In the longer run, breakthroughs in materials or batteries (aided by quantum simulations) could lead to better batteries in smartphones or more efficient solar cells, indirectly influencing our daily lives.
Industry transformation: Many industries will prepare now for future quantum disruption. Financial firms, for instance, are exploring quantum algorithms for portfolio optimization and risk analysis – even if real quantum advantage is years away, it will change how trading and finance software is built. Manufacturing and logistics companies are evaluating whether quantum annealing can improve supply chain optimization and scheduling. The energy sector is watching for new catalysts or materials that could result from quantum-aided discoveries (better fuel cells, cheaper solar panels, etc.). Intelligence and defense agencies are preparing for the need to secure data against future quantum attacks, as highlighted by government initiatives on post-quantum cryptography.
Future tech: Looking farther ahead, quantum computing is often mentioned alongside AI and biotechnology as one of the most disruptive technologies. Some foresee a synergy: quantum computers might help design more powerful AI models by optimizing or simulating aspects of neural networks. Others imagine a “quantum internet” where qubits are transmitted via entangled photons across fiber networks, enabling secure communications. Some even speculate about novel sensors or quantum-enhanced imaging in healthcare. While much of this is still science fiction, each year brings practical advances. Already, technology giants like Google say they are “about five years out” from having quantum systems that can run useful applications, according to media reports.
Education and workforce: The rise of quantum computing is creating new jobs and educational tracks. Universities are launching degree programs in quantum information science. Corporations (IBM, Microsoft, etc.) train employees and students via online labs and certifications. This cultural shift could lead to more tech-savvy graduates and a society more aware of advanced computing concepts. In this sense, quantum computing might be to computing what the internet was to communication: a fundamental new platform that eventually touches nearly every field.
Some experts caution that timelines are uncertain. Building error-corrected, large-scale quantum computers may still be a decade or more away. In the meantime, however, incremental progress adds value. For example, using a current device to solve a small chemistry problem or running a quantum-enhanced algorithm on the cloud provides insight and training that classical computers cannot match. From a strategic perspective, many companies view quantum computing research as essential long-term R&D. As Théau Peronnin put it, quantum is not an end goal itself, but a step change in the scale of computation – and pushing that scale could drive “better engineering” and growth across industries.
The Future of Quantum Computing
We stand at an exciting juncture. Quantum computing has moved out of pure theory and into experimental reality. Every few months, new milestones are announced: a higher qubit count, a demonstration of error correction, a quantum advantage proof-of-concept, or a new funding round by a startup. While hype abounds, so does genuine progress.
Looking forward, the community is focused on fault-tolerant quantum computing – creating logical qubits that can remain coherent indefinitely using quantum error correction. This will require many more physical qubits (possibly millions) but it is the key to scaling up. Parallel advances in quantum materials, control electronics, and cryogenics will support this.
For the average reader, what should they take away? First, quantum computing is not science fiction – it is a real, growing technology with significant investment (worldwide funding for quantum ventures reached billions of dollars in recent years). Second, its impact will likely be most visible in certain domains first (chemistry, encryption, optimization), rather than your personal laptop. But ultimately, quantum computing is poised to change our tech foundations. It may sound abstract when we talk about qubits and superposition, but the downstream effects – new medicines, faster internet security, AI breakthroughs – could be very concrete.
In my view, quantum computing exemplifies how science and engineering constantly push the boundaries of possibility. It invites us to prepare: students can start learning quantum programming today, companies can strategize for quantum-safe encryption and potential quantum-enabled products, and policymakers can fund education and standards (as NIST is doing for post-quantum cryptography).
Ultimately, quantum leap is an apt metaphor: quantum computing represents a jump to a new computational paradigm. It may take years or decades to fully mature, but when it does, the tech industry and society will experience a once-in-a-generation shift. The quantum era is coming, and the journey has already begun.
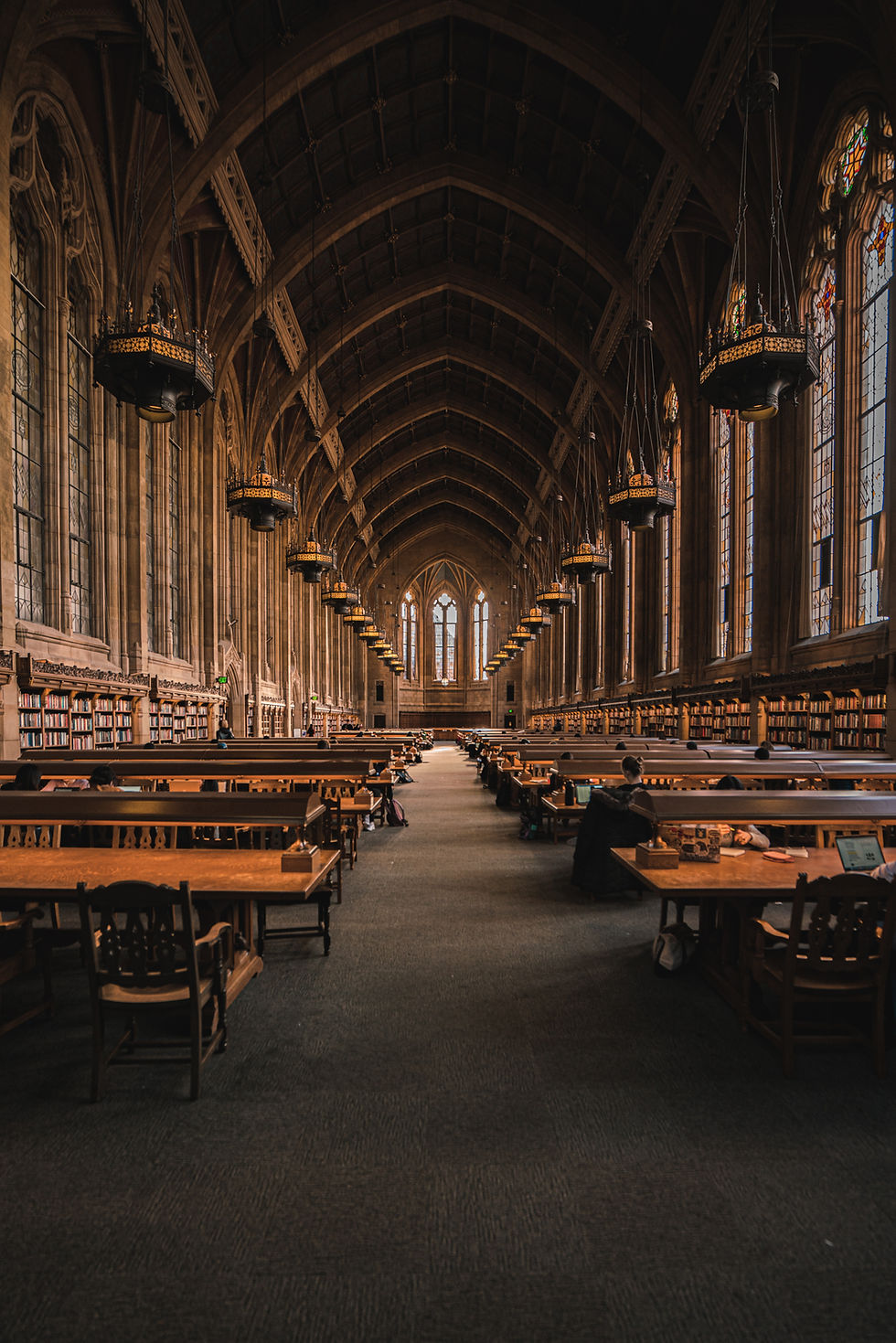
References
IBM ThinkPad: “What Is Quantum Computing?” (IBM) – IBM’s introduction to qubits and their types, including superconducting and trapped-ion qubits.
IonQ Quantum Cloud – “Pricing” (IonQ) – IonQ’s site explaining cloud access and academic credit ($10k).
Rigetti Computing Blog – “Quantum computing applications” (Rigetti) – Rigetti’s Azure Quantum sign-up (free $500 credit).
IBM Quantum (Website) – “Get started for free” (IBM) – IBM Quantum Platform offering 100+ qubit access with free monthly usage.
D-Wave Leap – “What Leap Customer Plans Are Available?” (D-Wave) – Explains Leap trial plan and LaunchPad.
IEEE Spectrum – “First Photonic Quantum Computer on the Cloud” – News on Xanadu’s cloud (8,12,24 qubits). Also notes photonic advantages.
Nature – “Quantum computational advantage with a programmable photonic processor” – Reports Xanadu Borealis boson sampling (9000 years vs 36 μs).
NIST – “What Is Post-Quantum Cryptography?” – Explains how quantum computers can break RSA (billions of years → days/hours).
McKinsey – “Blocking out the noise: Interview with a quantum computing expert” – CEO of Alice & Bob on quantum’s value and industries (AI, pharma, batteries).
McKinsey – “Pharma’s digital Rx: Quantum computing in drug R&D” – Discusses quantum’s potential for simulating molecules in drug discovery.
Comments