The Physics Behind Hypersonic Flight: Is the Future Already Here?
- Martin Low
- May 4
- 45 min read
Executive Summary
Hypersonic flight, defined as air travel at speeds above Mach 5 (over five times the speed of sound), is moving from the realm of research and military prototypes toward practical reality. This executive summary highlights the current state and significance of hypersonic technology in approximately 300 words.
Definition and Significance
Hypersonic flight occurs at velocities where traditional aerodynamic rules change – air molecules break apart (dissociate) due to intense frictional heating, creating extreme thermal and structural challenges. Overcoming these physics hurdles can unlock unprecedented travel speeds, cutting intercontinental trip times from hours to minutes. For military forces, hypersonic vehicles promise missiles or aircraft that maneuver unpredictably at speeds no defense system can easily intercept. For civilians, future hypersonic airliners or suborbital spaceplanes could revolutionize air travel, enabling same-day round trips across the globe.
Historical Context
The quest for ultra-fast flight dates back to the Cold War. Milestones like the X-15 rocket plane in the 1960s reached Mach 6.7, and aerospace engineers have been steadily pushing speed limits ever since. Until recently, hypersonic flight was limited to experimental craft and reentry from space. Today, however, we see a resurgence of high-speed innovation driven by both geopolitical competition and commercial ambition.
Current Innovations in Hypersonic Flight
In the military sphere, countries such as the United States, China, and Russia have prototype or deployed hypersonic missiles and glide vehicles, with Russia’s “Kinzhal” reaching roughly Mach 10 and China fielding the DF-17 glide vehicle. At the same time, aerospace companies and startups are investing in hypersonic propulsion (like scramjets) and advanced materials. Projects range from NASA and Boeing’s plans for a Mach 5 passenger jet by 2040 to SpaceX’s vision of suborbital point-to-point rocket flights.
Future Outlook
Experts forecast that some military hypersonic technologies will transition to civilian uses within the next two decades. Supersonic passenger travel is expected to return in the 2030s, and hypersonic or suborbital commercial flights could debut by the 2040s, fundamentally transforming global mobility and commerce. However, realizing this future will require addressing significant technical, ethical, and environmental challenges – from managing extreme heat to ensuring such advances benefit society at large and not only a select few.
In summary, hypersonic flight stands at the cutting edge of aerospace engineering. The “future” of flying at five times the speed of sound is fast approaching, with prototypes already streaking through the skies. The following sections delve into the physics behind hypersonics, their historical development, current breakthroughs, future possibilities, and the broader implications of a hypersonic age.
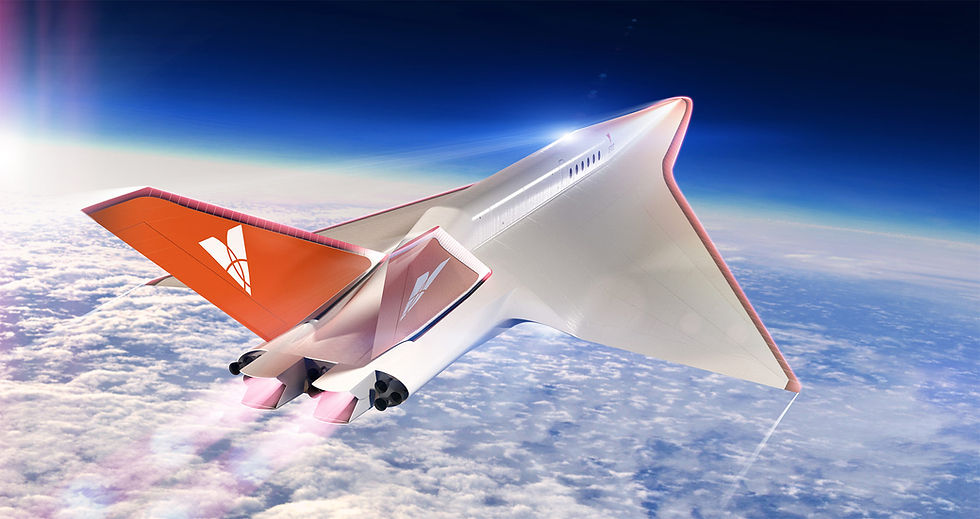
I. Introduction
In aviation, hypersonic flight refers to traveling through air at speeds of Mach 5 or greater – at least five times the speed of sound. At such velocities, the very physics of flight change. Air does not merely rush around a vehicle; it assaults it. The compression of air at a hypersonic vehicle’s leading edges produces intense shock waves, and the friction generates extreme heat capable of melting conventional materials. A hypersonic craft’s skin can soar to thousands of degrees, causing air molecules to ionize (lose electrons) in its wake. This regime poses unique engineering challenges in aerodynamics, propulsion, and materials science – challenges so daunting that for decades, sustained hypersonic flight seemed confined to research labs and science fiction.
Why is hypersonic flight relevant now? In recent years, a confluence of technological progress and global interest has thrust hypersonics into the spotlight. On the military front, major powers are engaged in what many call a hypersonic arms race. Reports of advanced missiles that can strike targets at Mach 10+ and evade defenses have captured public imagination and strategic concern. For instance, Russia has used air-launched “Kinzhal” missiles in conflict, weapons purported to reach around Mach 10, while China has tested and deployed medium-range missiles equipped with hypersonic glide vehicles that can maneuver at Mach 5–10. These developments suggest that the future is already here in military hypersonics – new systems are operational or on the cusp of being so, potentially redefining national security dynamics.
Simultaneously, commercial and civil aerospace sectors are revisiting high-speed flight for transportation. Nearly two decades after the retirement of the Concorde (the last supersonic airliner, cruising at Mach 2), a new crop of companies and agencies is dreaming even faster. Startups are designing passenger aircraft that could cruise at Mach 5, promising New York to Tokyo trips in a couple of hours instead of half a day. In 2018, for example, Boeing unveiled a hypersonic airliner concept – a sleek delta-wing design – that could fly at Mach 5 and cut transoceanic travel to two or three hours. NASA has likewise contracted studies on high-speed civilian transports that might enter service in the 2030s. Even the space industry has joined the conversation: SpaceX has floated the idea of using its Starship rockets to ferry passengers point-to-point on Earth, effectively offering suborbital hypersonic flights that could reach any city in under an hour.
The excitement is driven by both possibility and necessity. Global business and tourism hunger for faster travel options as air traffic grows, and a tech-savvy public raised on instant information now wonders if physical travel can approach the speed of digital communication. On the defense side, the prospect of missiles that can outrun and out-maneuver any interceptor has pushed governments to accelerate hypersonic research to avoid falling behind. Taken together, these factors have created a sense that a new era of flight – the hypersonic age – is imminent.
This article will explore the physics behind hypersonic flight and examine whether the much-anticipated future of ultra-fast air travel is finally arriving. We begin with a historical perspective on high-speed flight, then review current innovations by leading companies and government programs. We will peer into the future possibilities of hypersonic and even suborbital passenger travel, discussing expected timelines for military breakthroughs to trickle down into civilian life. Finally, we will consider the ethical and societal implications of hypersonic technology: its dual-use nature, environmental and safety challenges, and how international competition or cooperation might shape its development. The goal is a comprehensive, yet highly readable, survey of hypersonic flight – a topic where cutting-edge physics meets the real-world pursuit of “faster, higher, farther.”
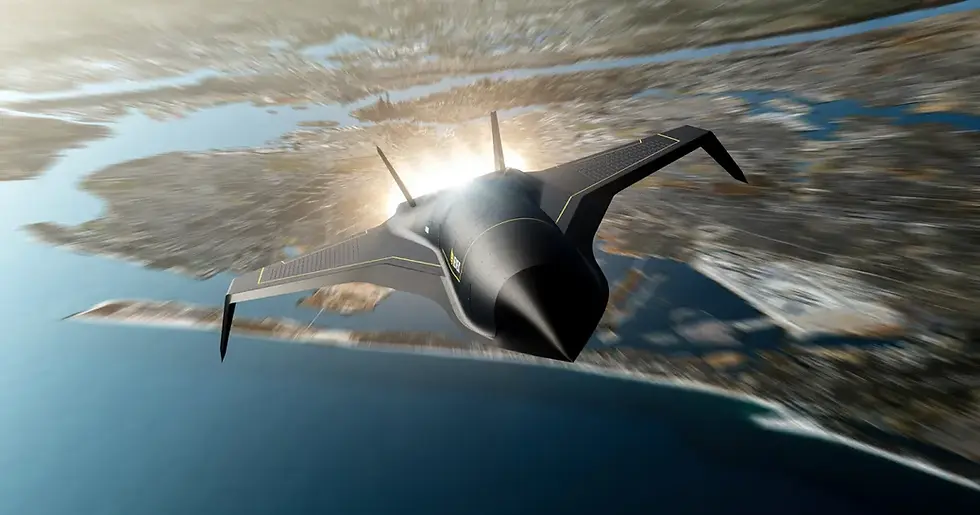
II. Historical Perspective
The dream of extreme speed in flight is almost as old as powered aviation itself. Breaking the “sound barrier” (Mach 1) was a monumental achievement in 1947, when test pilot Chuck Yeager’s rocket-powered Bell X-1 first exceeded Mach 1 in level flight. Yet engineers and futurists soon set their sights much higher – toward the hypersonic threshold of Mach 5 and beyond. To understand where we stand today, it is important to trace the key milestones in the development of high-speed flight, from early supersonic experiments to the verge of practical hypersonic vehicles.

From Supersonic to Hypersonic: The period after World War II saw rapid progress in high-speed aeronautics. By the late 1950s, military jets routinely flew at supersonic speeds (Mach 1–3), and aerospace engineers were planning experimental vehicles to venture into the hypersonic realm. A landmark achievement came in the early 1960s with the North American X-15 program – a rocket-powered research plane that remains a legend in aerospace history. The X-15, launched from a B-52 mothership, was essentially a piloted missile with tiny wings. In 1967, it set an unofficial speed record for atmospheric flight by reaching Mach 6.7 (approximately 7,200 km/h) at the edge of space. Piloted by U.S. Air Force Major William “Pete” Knight, the X-15’s record still stands over half a century later for winged aircraft. The image below shows an X-15 in flight during this era, illustrating the rocketplane design needed to achieve hypersonic speeds in the 1960s.
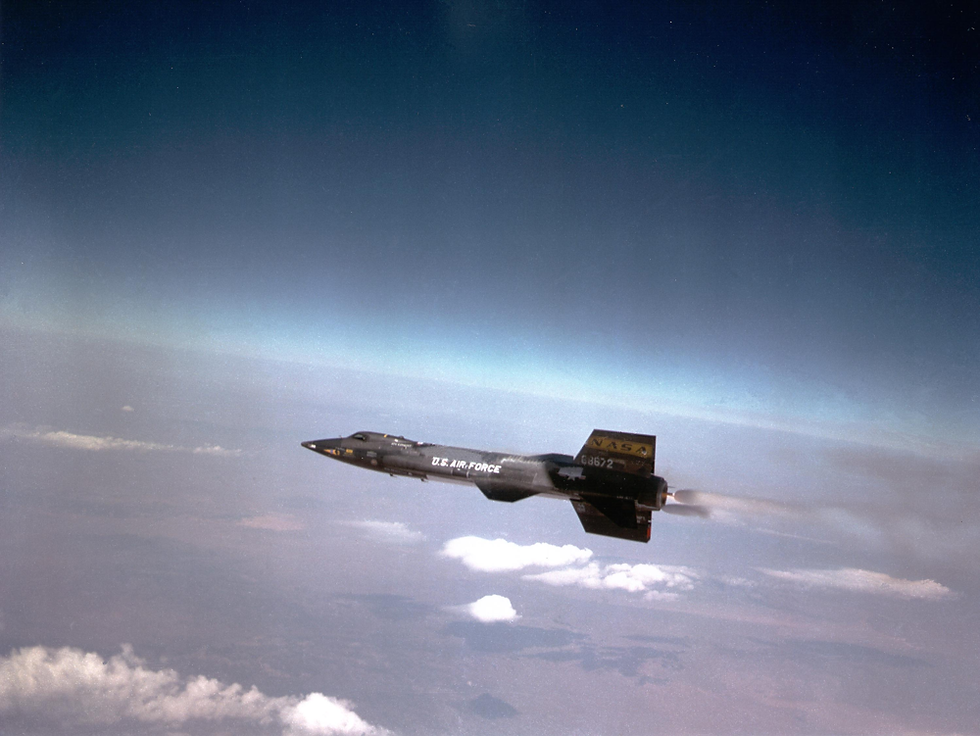
An X-15 hypersonic research aircraft in flight (1960s). The X-15 was air-dropped from a B-52 bomber and ignited its rocket engine to sprint past Mach 6, yielding invaluable data on hypersonic aerodynamics and heating. It demonstrated that piloted hypersonic flight was possible, albeit for brief periods, laying groundwork for future spaceplanes.
The X-15 program gave scientists practical experience with phenomena like extreme aerodynamic heating (its pilots observed the craft’s exterior glowing red-hot). It also underscored the limits of 1960s technology – each flight was a short rocket burn followed by glide back to Earth, and the vehicle’s skin required special heat-resistant materials and even ablative coatings (materials that slowly char away to carry heat off) for the fastest flights. The knowledge gained would later inform the design of reentry capsules and the Space Shuttle.
Space Race contributions: In parallel with atmospheric efforts, the dawn of human spaceflight in the 1960s meant that, by definition, every returning astronaut became a hypersonic traveler. When Yuri Gagarin became the first man in orbit in 1961, his Vostok capsule tore back into the atmosphere well above Mach 5 – making him the first human to experience hypersonic speeds (albeit inside a protected capsule). The same year, American astronaut Alan Shepard’s suborbital flight reentered at hypersonic velocity. These feats weren’t sustained atmospheric flights, but they proved objects (and people) could survive transit through the hypersonic regime using blunt-shaped vehicles that created shock waves to keep most heat away (a technique proposed by engineers H. Julian Allen and Alfred Eggers). By the mid-1960s, nuclear warheads on ballistic missiles were routinely traveling at Mach 15–20 during reentry – technically hypersonic, but on predictable ballistic paths. The military interest grew in making those warheads or vehicles maneuverable at hypersonic speeds, to evade defenses. Early concepts like the American Dyna-Soar spaceplane (cancelled in 1963) and Soviet research into boost-glide weapons sowed the seeds for what would much later emerge as operational hypersonic glide vehicles.
Concorde and the limits of supersonic transport: While government research pushed the envelope, civilian air travel also edged faster. The Anglo-French Concorde and the Soviet Tu-144 were 1970s marvels that cruised at around Mach 2, ferrying passengers at twice the speed of sound. Concorde’s Mach 2.04 cruising speed halved transatlantic flight times and proved there was at least some demand for faster-than-sound travel. However, Concorde never approached hypersonic speeds, and its eventual 2003 retirement marked the end of commercial supersonic flight – until a new generation of designs can succeed. Concorde’s story highlighted hurdles that any high-speed transport (especially one aiming for hypersonic speeds) must overcome: high fuel consumption, stringent structural demands, exorbitant development costs, and environmental or regulatory challenges (Concorde was banned from supersonic flight over land due to sonic boom noise).
The long hiatus: During the late 20th century, hypersonic research progressed fitfully. The U.S. in the 1980s launched the ambitious National Aerospace Plane (NASP) program, which aimed to build a single-stage-to-orbit vehicle powered by a scramjet (supersonic combustion ramjet) engine. The NASP (also known as the X-30) never got beyond the drawing board due to technical complexity and budget issues, but it spurred advances in high-temperature materials and computational fluid dynamics. Meanwhile, unmanned hypersonic vehicles achieved notable successes. In 1980, the Soviet Union flew “Kholod,” a hydrogen-fueled scramjet test article, to about Mach 6. And in the early 2000s, NASA’s Hyper-X program made history with the X-43A – a small uncrewed vehicle that became the first air-breathing aircraft to fly at hypersonic speeds. In 2004, the X-43A accelerated under scramjet power to Mach 9.6, roughly 7,000 mph at 110,000 feet. It was a brief test (the engine burned for about 10 seconds), but enough to set a world record for jet propulsion. A follow-on, the Boeing X-51 “Waverider,” in 2013 sustained a scramjet burn for a few minutes, cruising at Mach 5.1 before plunging into the ocean. These experimental flights in the 2000s proved that hypersonic propulsion could work – that an engine could gulp air and propel a vehicle at five to ten times the speed of sound – but they were essentially bullets shot through the sky, not practical vehicles.
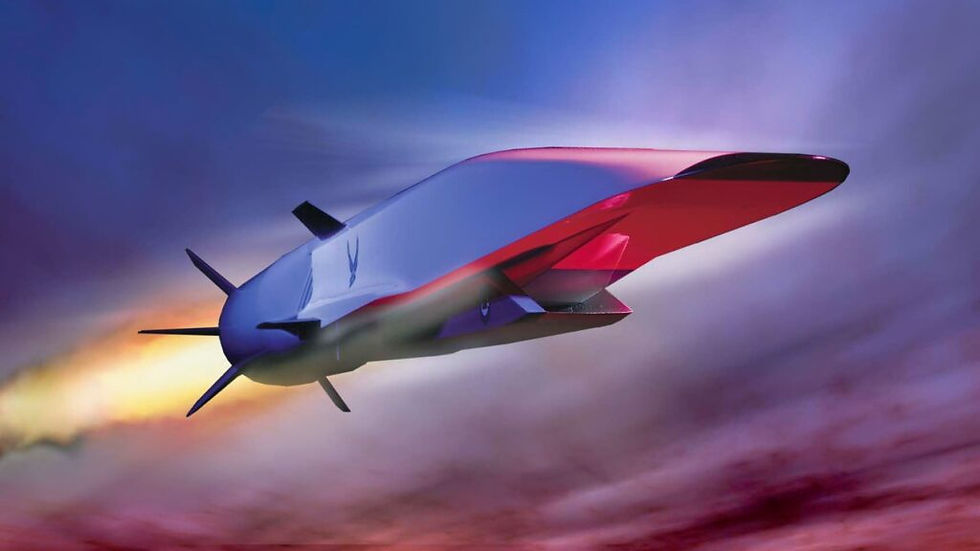
Renewed focus in the 21st century: After a lull, the 2010s saw hypersonic research surge forward, driven by both military urgency and enabling technologies. Computational modeling and simulation tools became far more powerful, allowing engineers to understand hypersonic fluid dynamics and heating in greater detail. Materials like carbon–carbon composites (first used on the Space Shuttle’s leading edges) and new metal alloys began to offer better heat tolerance. And as we entered the 2020s, global strategic developments – particularly tests by Russia and China of new hypersonic weapons – injected fresh momentum (and funding) into hypersonic projects. Governments dusted off old concepts of maneuverable reentry vehicles and hypersonic cruise missiles, this time aided by modern electronics, guidance systems, and manufacturing techniques (like 3D-printing of complex engine parts).
In summary, the journey to hypersonic flight has been long and nonlinear. We went from the experimental rocket planes of the 1960s, to a period of maturation and occasional breakthroughs (often tied to space efforts), and now into a new phase where what was once the province of rare feats and stunts is edging closer to practical application. The historical lessons – from the X-15’s heroic flights to Concorde’s operational challenges – continue to inform today’s hypersonic engineers. With this context, we now turn to the present day: What hypersonic innovations are happening right now? Who are the players leading this new era, and what have they achieved so far?
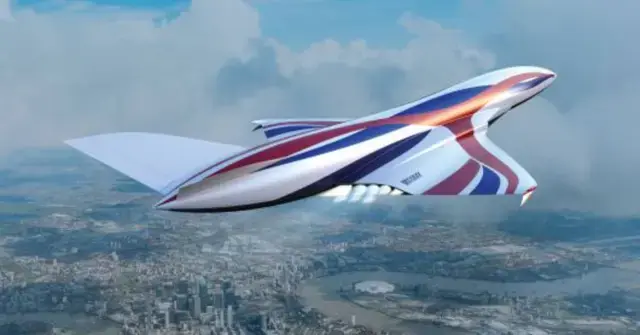
III. Current Innovations
Hypersonic technology is experiencing a renaissance in the 2020s. Several cutting-edge projects – both military and civilian – have recently reached major milestones, heralding the arrival of practical hypersonic flight. In this section, we discuss the latest developments in hypersonic vehicles and systems, highlighting key companies, countries, and programs at the forefront. Innovations range from experimental aircraft and missiles that have demonstrated Mach 5+ performance, to prototypes of passenger planes that might one day make hypersonic travel routine. We’ll examine both military applications (which currently lead the field) and commercial efforts, since progress in one often catalyzes breakthroughs in the other.
Military Hypersonic Flight Programs and Prototypes
Military interest in hypersonics is driven by the desire for game-changing capabilities: missiles that strike faster or farther, and vehicles that can penetrate defenses through sheer speed and maneuverability. As of 2025, at least three major powers – the United States, Russia, and China – have active hypersonic weapon programs, with a few systems already deployed or very close to deployment.
United States: The U.S. has several hypersonic projects underway, reflecting a diversified approach. One class of weapons is the boost-glide vehicle: a rocket boosts a payload to high altitude and speed, then the payload (a maneuverable glider) coasts and maneuvers at hypersonic speed toward its target. The U.S. Army and Navy are co-developing a Common Hypersonic Glide Body under programs named Long-Range Hypersonic Weapon (LRHW for the Army) and Conventional Prompt Strike (for the Navy). These are intended to be fielded in the mid-2020s as ultra-fast conventional strike options. Another approach is the air-launched hypersonic missile. The Air Force tested the AGM-183A ARRW (Air-launched Rapid Response Weapon) – a boost-glide system launched from a B-52 bomber. In 2022 and 2023, ARRW prototypes did achieve high speeds, but the program encountered test anomalies and has been scaled back, illustrating the challenges of making such systems reliable. More promising have been U.S. efforts on air-breathing hypersonic cruise missiles. In 2021–2022, DARPA’s HAWC (Hypersonic Air-breathing Weapon Concept) program successfully flew scramjet-powered test vehicles. In its final flight in January 2023, a HAWC vehicle, after being dropped from a B-52, ignited a booster and then its scramjet. It flew over 300 nautical miles at above Mach 5, reaching altitudes over 60,000 feet – meeting all test objectives. This marked the first time a scramjet-powered weapon demonstrated prolonged hypersonic cruise, and it has paved the way for a new prototype missile called HACM (Hypersonic Attack Cruise Missile). The HACM, a project awarded to Raytheon and Northrop Grumman, aims to deliver an operational air-launched hypersonic missile to the U.S. Air Force by around 2027. It will use an improved scramjet engine (built by Northrop) to reach speeds possibly up to Mach 10, giving fighter jets or bombers a formidable standoff weapon. The U.S. is also exploring hypersonic aircraft beyond missiles – notably the rumored Lockheed Martin SR-72, a reconnaissance/strike drone concept nicknamed “Son of Blackbird.” The SR-72 is envisioned as a turbine-based combined-cycle aircraft (turbines for lower speeds, transitioning to ramjet or scramjet for Mach 6+), potentially capable of intelligence missions at hypersonic speeds. Though largely classified, Lockheed has hinted that hypersonic tech is sufficiently mature to begin work on a Mach 6 demonstrator. In short, the U.S. finds itself in an aggressive R&D phase: it has no deployed hypersonic weapons as of 2025, but multiple prototypes in advanced testing, and a clear goal to field both glider and scramjet systems within a few years.
Russia: Russia has been particularly vocal about its hypersonic weapons, even claiming a world-first by deploying some of them operationally. In 2018, President Vladimir Putin unveiled a suite of “next-generation” strategic weapons, prominently featuring the Avangard hypersonic glide vehicle. Avangard is launched atop an ICBM and can reportedly reach about Mach 20 during its guided descent. In late 2019, Russia announced Avangard was in service on a limited number of SS-19 ICBMs, making it likely the first deployed hypersonic glide weapon. Russia also developed the Kinzhal (Kh-47M2), an air-launched ballistic missile modified from the Iskander short-range missile. Fired from a high-speed MiG-31K jet, the Kinzhal accelerates to roughly Mach 10 and can carry either conventional or nuclear warheads. Russia has used Kinzhals in the ongoing conflict in Ukraine, marking the first combat use of hypersonic missiles. Another Russian system, the 3M22 Zircon (Tsirkon), is a ship- or submarine-launched hypersonic cruise missile. Unlike gliders or ballistic Kinzhals, Zircon uses a scramjet engine and flies within the atmosphere. Test launches have been successful, with Russian officials stating Zircon hit speeds of “more than Mach 8” in trials. By 2022, Russia announced the first deployment of Zircon missiles on a naval frigate, making it likely the world’s first operational hypersonic cruise missile. These achievements, if taken at face value, position Russia as a leader in fielding hypersonic weapons. However, Western analysts note that systems like Kinzhal are essentially aeroballistic missiles (fast, but following mostly ballistic trajectories), and that Russia’s small number of launch platforms limits their strategic impact. Still, there is no doubt Russia’s investments (including plans for a high-speed successor to the Kh-95 long-range missile, and a hypersonic version of its upcoming Sarmat ICBM) have raised the bar and spurred competition.
China: China has rapidly advanced its hypersonic capabilities in the past decade, to the point that U.S. defense officials now assess that China is leading in some areas of hypersonic weapons development. Beijing’s most prominent system is the DF-17, a medium-range ballistic missile mated with a hypersonic glide vehicle (HGV) known as the DF-ZF. The DF-17 can carry the HGV to a launch speed above Mach 5, after which the glide vehicle separates and maneuvers toward its target at speeds in the Mach 5–10 range. Chinese state media unveiled the DF-17 during a military parade in 2019, and it is believed to be deployed with the People’s Liberation Army Rocket Force, giving China an operational regional hypersonic strike asset. Beyond that, China startled the world with a test reported in August 2021: it launched what U.S. officials described as a fractional orbital bombardment system with a hypersonic glider. In that test, a rocket lifted a glide vehicle into low-earth orbit; the vehicle then reentered and circumnavigated the globe before descending on a target (reportedly missing by a couple of dozen miles). This demonstration of a long-range, orbit-capable hypersonic system led a U.S. general to liken it to a “Sputnik moment,” underscoring how advanced China’s program had become. In addition to gliders, China has worked on scramjet-powered vehicles. In 2018, it tested the Starry Sky-2 (Xingkong-2), a experimental waverider vehicle boosted to high altitude that then flew on scramjet power at Mach 6+. Chinese sources claimed Starry Sky-2, which they termed a “nuclear-capable” hypersonic prototype, was a success. China also reportedly has multiple hypersonic wind tunnels (at least 20 facilities) that can test up to Mach 8–12 conditions, far more than any other nation’s capacity, giving it a strong R&D base. All these efforts indicate that China is integrating hypersonic weapons into its strategy – particularly for regional scenarios in the Asia-Pacific, where such weapons could threaten U.S. carrier strike groups or bases with little warning. Like Russia, China appears to be focusing both on boost-glide systems and on air-breathing cruise missiles, aiming for a diverse arsenal.
Other Countries: Several other nations have initiated hypersonic programs, often in collaboration with allies:
India has worked with Russia on the BrahMos-II project (a successor to the BrahMos supersonic cruise missile) targeting Mach 7, and it tested its own HSTDV (Hypersonic Technology Demonstrator Vehicle) in 2020, achieving about 20 seconds of scramjet-powered flight at Mach 6. India’s goal is to develop a hypersonic cruise missile within this decade.
France is leading a European effort: it successfully tested a hypersonic glider prototype called V-MaX in June 2023 (launched on an sounding rocket, it validated materials and control at high speed). France, together with Germany, also launched a project under NATO to study hypersonic technology and defenses. Europe’s aerospace industry (Airbus, MBDA, etc.) is exploring high-speed propulsion, though Europe has yet to field a specific weapon system.
Japan and Australia are also contributing. Japan is developing the HVGP (Hyper Velocity Gliding Projectile) for anti-ship and anti-landing defense (aiming for deployment in the late 2020s), as well as a scramjet-powered cruise missile for the 2030s. Australia, partnering with the U.S., ran the HIFiRE series of hypersonic flight experiments and is now part of SCIFiRE, a cooperative project with the U.S. to prototype an air-breathing hypersonic missile (which might evolve into a system similar to HACM).
In summary, the military landscape features multiple programs bearing fruit. Russia and China have stolen a march by fielding some hypersonic weapons already, while the U.S. and allies are pressing hard to test and deploy their own within a few years. Notably, all these developments benefit from and contribute to the broader knowledge pool of hypersonic flight – data from military tests (e.g., on thermal protection or guidance at Mach 8) can inform civilian research, and vice versa. Indeed, much of the current innovation is happening in defense agencies and companies (Lockheed Martin, Raytheon, Northrop Grumman, China’s CASC, Russia’s NPO Mashinostroenia, etc.), which serve as the tip of the spear in hypersonic R&D.
Commercial and Civilian Efforts
While the military pushes the envelope in weaponry, an equally important question looms: Will hypersonic technology jump from missiles to passenger aircraft or other civil applications? A number of companies – from startups to aerospace giants – are actively working on making hypersonic (or at least high-supersonic) travel a reality for civilian transport. These efforts are driven by the tantalizing promise of drastically reduced travel times for passengers and cargo. However, they face steep challenges, including engineering complexity, regulatory hurdles, and environmental concerns. Here we highlight some of the promising projects and players in the commercial hypersonic arena:
Boom Supersonic and the Return of Supersonic Jets: Before diving into full hypersonics, it’s worth noting the resurgence of supersonic passenger flight as a stepping stone. Denver-based startup Boom Supersonic is developing the Overture, a 65-88 passenger airliner designed to cruise at about Mach 1.7. Several major airlines (United, American, Japan Airlines) have placed pre-orders, and Boom aims to fly Overture by the late 2020s (targeting 2029 for entry into service). While Overture is not hypersonic, Boom’s work is rekindling interest in high-speed aerodynamics, materials, and engines. The company is also building a demonstrator called XB-1 to test technologies. Importantly, Boom and others are grappling with sonic boom mitigation and fuel efficiency – issues that only intensify as one approaches Mach 5. v is another notable project here: it’s a supersonic X-plane designed to produce a much softer sonic “thump.” Scheduled to fly in 2023–2024, the X-59’s data might lead to lifting the ban on supersonic overland flight, which would greatly favor any future hypersonic transport (since a Mach 5 airliner would also produce booms). In essence, Boom Supersonic, Aerion (before it folded in 2021), Spike Aerospace, and others in the supersonic jet realm are laying groundwork that higher-speed projects can build upon, particularly in aerodynamics and regulatory acceptance.
Hermeus and Hypersonic Airliners: One of the most ambitious startups directly targeting hypersonic passenger travel is Hermeus, based in Atlanta. Founded in 2018, Hermeus has set out a plan to develop a Mach 5 capable airplane that could serve both military and eventual commercial roles. Their approach is to use a turbine-based combined cycle (TBCC) engine called “Chimera.” This engine essentially pairs a modified turbojet (for low speeds) with a ramjet (for high speeds), using a common inlet and exhaust. In 2022, Hermeus successfully tested the Chimera engine, transitioning from turbojet mode to ramjet mode – a critical demonstration for Mach 5 propulsion. The company is first building a small unmanned demonstrator called Quarterhorse to validate the engine and other systems. Impressively, Hermeus has attracted U.S. Air Force support: the Air Force awarded the company a contract (worth about $60 million) to adapt its concept into a hypersonic executive jet for defense VIP transport (sometimes playfully dubbed a potential “Air Force One” for the future). The roadmap envisions a subsequent prototype called Darkhorse (possibly a drone or optionally piloted vehicle) and ultimately a commercial 20-seater jet named Halcyon capable of New York-to-London in 90 minutes. While these goals are bold, Hermeus is one of the few ventures that is actually building and testing hardware with hypersonic performance in mind. If successful, it could become the SpaceX of hypersonic aviation, dramatically reducing the time and cost to develop ultra-fast aircraft. Their progress also suggests that military-civil fusion: initial funding and use by the military (for high-speed reconnaissance or transport) could lead to a later civilian airliner design, aligning with the historical pattern of new aviation tech.
Reaction Engines and SABRE: In the UK, Reaction Engines Ltd made headlines with its revolutionary SABRE (Synergetic Air-Breathing Rocket Engine), a hybrid engine intended for a spaceplane called Skylon. SABRE is designed to act like a jet at low altitude and like a rocket at high altitude. Key to SABRE is a lightweight pre-cooler heat exchanger that can take incoming air (at thousands of degrees from ram compression at Mach 5) and cool it to manageable temperatures in fractions of a second. In tests, Reaction Engines demonstrated this pre-cooler at conditions simulating over Mach 5 flight. The SABRE engine promises to enable an aircraft to take off from a runway, accelerate to Mach 5 in the atmosphere using oxygen from the air, then transition to rocket mode to reach orbit – a truly transformative capability. Even for point-to-point hypersonic travel, a SABRE-based vehicle could be highly efficient. However, Reaction Engines hit financial troubles in 2023–2024. After decades of R&D and some government support, the company struggled to secure enough private investment to move to full-scale development and had to downsize or fold its projects. Despite this setback, the SABRE technology is proven in principle and remains one of the most innovative approaches to hypersonic propulsion. It’s possible that a larger aerospace entity or government will pick up Reaction Engines’ work. If SABRE or a similar concept succeeds, it could enable reusable hypersonic vehicles that operate at a fraction of current launch costs – a game-changer for both high-speed transport and space access.
SpaceX and Suborbital Travel: Although primarily focused on orbital rockets, SpaceX has pitched the idea of using its heavy Starship rocket for Earth-to-Earth transportation. In this concept, passengers would board a rocket in, say, New York, launch briefly into space, and reenter to land in Shanghai in under an hour. Technically, this is hypersonic flight – in fact, faster than Mach 20 during reentry. In 2017, SpaceX released a flashy video of this vision, and by 2022 the U.S. military had invested in a small contract with SpaceX to study using Starship for rapid global cargo delivery. The Department of Defense calls it “Rocket Cargo” – envisioning delivering 100 tons of matériel anywhere on Earth quickly. As of 2025, Starship has not yet reached orbit, and many skeptics point out the enormous regulatory, safety, and cost issues of using giant rockets for routine earthly travel (imagine the sonic booms and the launch pad requirements in city centers). However, SpaceX’s concept has stirred discussion about the ultimate in hypersonic travel: suborbital trajectories that cut globe-spanning trips to under 1–2 hours. Even if Starship itself doesn’t become a passenger craft, the idea stands as the upper end of hypersonic ambition. It highlights that rocket technology and hypersonic aviation are converging – reusable rockets like Starship essentially are hypersonic vehicles (on reentry) that could be adapted for point-to-point use with enough demand and development of infrastructure.
Other Notable Players: A few more companies and initiatives deserve mention:
Stratolaunch – the company known for building the world’s largest airplane – has repurposed itself as a hypersonic testing service. It has developed Talon-A, an unmanned hypersonic testbed vehicle that can be air-launched from its giant carrier plane. Talon-A is a rocket-powered glider intended to fly multiple missions testing hypersonic technologies (avionics, materials, etc.) and then glide to a runway landing. This could provide valuable flight test opportunities for researchers outside of government programs.
Destinus – a European startup (based in Switzerland) – is developing a hydrogen-fueled unmanned hypersonic drone for cargo, with long-term vision of a passenger version. They’ve built subscale prototypes and intriguingly plan to use liquid hydrogen not only as fuel but also as coolant for a propulsive cooling scheme.
University and Government Labs: Around the world, many universities and agencies continue fundamental research. For example, Australia’s University of Queensland was instrumental in scramjet tests (HIFiRE and others). In the US, NASA and the Air Force Research Laboratory are experimenting with new materials (like ultra-high-temperature ceramics), and with novel engine cycles (like rotating detonation engines) that could find use in future hypersonic propulsion. Such research is often done in parallel with big development programs, feeding new discoveries into the pipeline.
Overall, the current state of hypersonic innovation is vibrant and multi-faceted. We see military prototypes hitting their marks – for instance, a scramjet cruise missile demonstrator flying successfully at Mach 5 – which builds confidence in the underlying physics and engineering. We also see commercial players taking those lessons and pushing toward applications that directly touch people’s lives (fast travel). A noteworthy point is that many of these efforts are interconnected: Boeing, for example, is involved in military programs and also envisioning civil hypersonic jets; likewise, materials developed for a SpaceX Starship heat shield might inform how to build a hypersonic airliner’s nose.
Crucially, the support of governments and large institutions is aiding even the commercial projects. NASA is funding research into high-speed passenger concepts with an eye on service entry in the 2030s. The U.S. Air Force is seeding startups like Hermeus through contracts. This mirrors the origin of jet aviation where military investment eventually led to the Boeing 707 and modern airliners. It suggests that some of today’s bleeding-edge tech (initially too expensive or risky for airlines) might gradually transition to civilian use as it matures and as economies of scale develop.
Now that we’ve reviewed who is leading and what has been achieved up to now, let’s look ahead. What do experts predict about when supersonic and hypersonic travel might become commonplace? What future possibilities emerge if these technologies succeed, and how might they impact society at large?
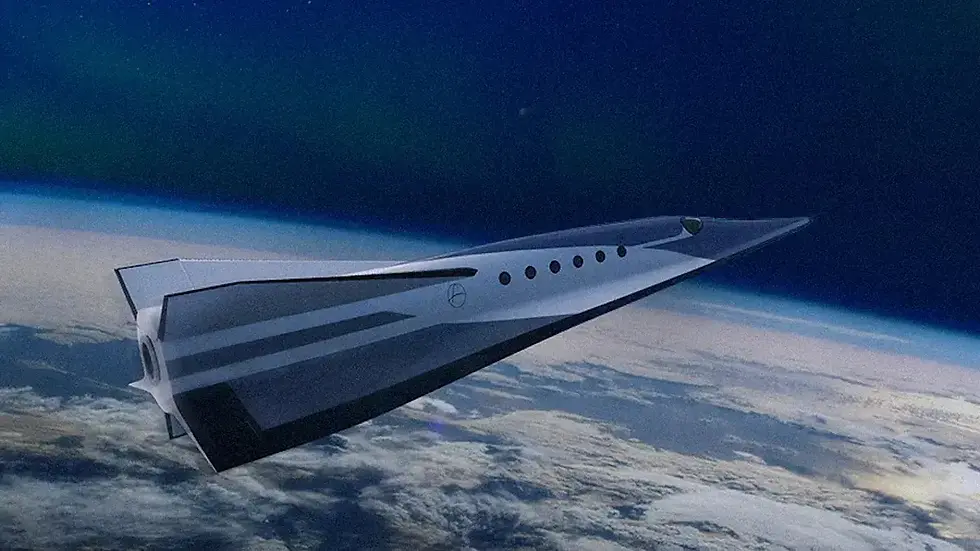
IV. Future Possibilities
The trajectory of hypersonic flight innovation points toward a future that would have seemed like science fiction just a few decades ago. Imagine traversing the globe in a few hours, or launching into space from a runway, or deploying critical cargo across continents at lightning speed. Such scenarios are the promise of hypersonic and ultra-high-speed flight. In this section, we will explore forecasts and expert insights on what might be coming in the next decades. We will consider timelines for different domains – supersonic resurgence, hypersonic passenger travel, and suborbital spaceflights – and discuss the potential impacts if these become reality. While predicting the future is always fraught with uncertainty, current trends and stated plans give us a reasonable sketch of what to expect through the 2030s and 2040s.
Supersonic Comeback (2020s–2030s): In the near-term, the most concrete developments will be the return of civilian supersonic aircraft. As noted, Boom Supersonic’s Overture airliner is slated for introduction around 2029. If Boom succeeds, by the early 2030s we could see limited but regular supersonic routes (likely over oceans to avoid boom issues) serving business travelers and premium leisure markets. Other companies or even legacy aerospace firms may follow suit with their own designs – for instance, there are concept sketches of business jets that fly just over Mach 1 to save time without causing sonic booms. By 2035, it’s plausible that a handful of supersonic aircraft models will be in operation, essentially picking up where Concorde left off but with modern efficiency and hopefully somewhat broader use. Notably, NASA’s X-59 project (with Lockheed Martin) is expected to produce data by the mid-2020s that may allow regulators to certify “low boom” supersonic jets for overland flight. If that happens, the market for supersonic travel could greatly expand (imagine a network of supersonic flights crisscrossing the U.S. or Europe-Asia in half the time of today’s jets). Experts forecast that by 2040, mid-sized supersonic passenger planes could be offering business-class service on key routes. These would still mainly cater to affluent travelers given higher ticket prices (Boom has mentioned ~$5,000 round-trip across the Atlantic), but they mark a step change in air travel speed.
Hypersonic Passenger Flights (2030s–2040s): When it comes to true hypersonic (Mach 5+) passenger travel, timelines stretch further out, but serious groundwork is being laid now. NASA and Boeing have publicly discussed a goal of a hypersonic commercial aircraft by around 2040. This is supported by contracts like the one NASA awarded in 2023 to Boeing and Northrop Grumman to develop concepts for Mach 3–5 airliners that could enter service in the 2030s. The implication is that a prototype or demo could fly in the early 2030s, leading to a passenger-carrying hypersonic jet about a decade later. Boeing’s own vision, revealed in 2018, imagined a Mach 5 airliner in operation by 2040 – a timeframe that aligns with these NASA studies. Experts emphasize that to meet that schedule, several challenges need resolution in the 2020s: identifying materials that can withstand temperatures over 3,000 °F (≈1650 °C) continuously, developing engines that can operate efficiently from standstill to Mach 5, and addressing economic factors (no airline will buy a Mach 5 plane that isn’t reliable and somewhat affordable to run). Progress is visible on the materials front, with research into ceramic matrix composites and new titanium alloys for airframe skins. On engines, the TBCC approach (turbine combined with ramjet) that Hermeus and others are pursuing is likely the favored solution for the first hypersonic airliner, because pure scramjets – while faster – are still less proven for controlled passenger flight. Hermeus’ timeline is aggressive: they claim they might fly a small hypersonic passenger-capable jet before 2030. If that happened (even as a tech demo), it would greatly accelerate broader development. More conservative forecasts from aviation analysts suggest 2040–2050 as the window when we might see the first regularly scheduled hypersonic air passenger service. Initially, such service would likely be limited to niche routes and extremely high-end travelers (much as the Concorde was), but it could expand if costs drop over time. Imagine a future where a network of Mach 5 jets connects major global hubs: one could depart London after breakfast and arrive in Sydney by afternoon local time, effectively shrinking the planet.
The impact of hypersonic passenger travel would be profound. Transcontinental business could be conducted almost in real-time; for instance, executives could attend meetings across oceans and be back home the same day. Tourism might change with far-flung destinations becoming feasible for shorter trips. There could be “hypersonic airlines” offering premium services much like today’s private jet charters but on steroids. However, widespread adoption would also depend on solving environmental concerns (more on that in the next section) – these planes would need to be as green as possible or use sustainable fuels to avoid backlash in a climate-conscious era.
Point-to-Point Suborbital and Cargo (Late 2020s–2040): On the military and perhaps logistics side, using rockets or hypersonic gliders for ultra-fast cargo delivery is being actively explored. The U.S. military’s interest in rocket-based global logistics suggests that by the late 2020s, we might see a demonstration of a point-to-point SpaceX Starship flight delivering a payload thousands of miles away in under an hour. Indeed, the U.S. Air Force has a program aiming for a test of this capability around 2025–2026. If those tests succeed, it could pave the way for emergency cargo services – think disaster relief supplies or critical spare parts delivered via a controlled reentry vehicle. By the 2030s, if space launch costs continue to drop and reusability improves, one could envision specialized fleets of suborbital transports for ultra-urgent deliveries (perhaps operated by companies like SpaceX, Blue Origin, or new entrants). Widespread civilian passenger use of such rockets is harder to predict. Elon Musk’s bold claims aside, launching people on ballistic trajectories comes with safety and comfort challenges (e.g., very high G-forces on launch and reentry, and the perception of risk similar to spaceflight). It is likely that passenger hypersonic travel will initially favor plane-like vehicles (winged craft taking off from airports) over ballistic rockets, simply because of the existing aviation infrastructure and regulatory framework. However, in the 2040s and beyond, if companies demonstrate impeccable safety records for rocket travel, specialized routes (such as New York to Shanghai in 40 minutes) might emerge for those willing to pay and strap in. One could think of it as the ultimate extension of first-class: extremely fast, expensive, and requiring a bit of astronaut-style tolerance.
Military-Civilian Tech Transition: A critical aspect of these future timelines is how quickly military innovation will feed the civilian sector. Historically, many revolutionary aviation technologies saw about a 10-20 year gap between military debut and civilian adoption (for example, jet engines were first used in WWII fighters in the 1940s, and by the late 1950s they powered commercial airliners; GPS was military-only in the 1980s and widely civilian by 2000s). If this pattern holds, one might expect that since military hypersonic systems are appearing in the early 2020s, civilian spinoffs (like passenger craft) could appear by the 2040s. This aligns with the aforementioned estimates. Some specific transitions could include:
Propulsion: The scramjet engines proven on missiles like HAWC or on India’s HSTDV will inform the design of the engines for aircraft. A military scramjet that today runs for 5 minutes on a missile might evolve into a longer-running engine for a drone or transport by mid-2030s.
Materials: High-temperature composites used in boost-glide warheads or hypersonic glider heat shields (designed to survive Mach 20 reentry) might find their way into leading edges and nose cones of future airliners, enabling them to sustain Mach 5 for hours. Similarly, cooling techniques (like transpiration cooling, where coolant is bled through pores to the hot surface) being studied for gliders could apply to civilian craft.
Design Tools: The advanced computer simulations and wind tunnel test data coming from current classified programs will improve the knowledge base for all designers. By the time a company builds a hypersonic airliner, they will benefit from perhaps 20+ years of accumulated research data from various missile tests and X-planes.
Infrastructure and Expertise: As militaries train engineers and pilots (or operators) for hypersonic vehicles, some of that expertise will eventually flow to the private sector. For instance, the Air Force might have its test pilots or drone operators proficient in flying boost-glide vehicles or high-speed drones – experience that could help regulators certify civilian hypersonic pilot training programs down the road.
Given these considerations, many aerospace analysts predict the early civilian hypersonic operations will likely be in partnership with government agencies (similar to how early jet airliners often had backing from military orders or technology). A likely scenario: a company builds a hypersonic business jet with partial funding from a military (for dual-use as a recon aircraft), it gets proven in governmental service by late 2030s, and then an airline or private company begins limited commercial use around 2040.
Potential Impact: If and when hypersonic travel becomes reality, the effects will be far-reaching:
Economic: The world could see an even more accelerated globalization of business. Time zones and distance might become less of a barrier for commerce. High-value industries (finance, tech, elite services) could cluster even more around global hubs connected by hypersonic routes. There could also be a stratification in travel – a new tier above first class, accessible only to the ultra-wealthy or on urgent business, creating perhaps a larger gap in experience between those who can afford such travel and those who cannot.
Transportation Networks: Hypersonic point-to-point might start eroding the dominance of long-haul subsonic airliners in certain segments. Just as high-speed rail took some market share from airlines for medium distances, hypersonic craft could take the top layer of long-distance travel. However, it might also create new demand – people may travel more often or further when travel times are cut dramatically.
Societal and Cultural: Culturally, the world could feel “smaller” yet again. Previously remote regions might become viable for day trips. For example, one could attend a conference in Sydney and be back in Los Angeles the same day, which is impossible now. This could enhance cross-cultural exchanges for those with access, but might also raise concerns about environmental footprint.
Space Access: If technologies like SABRE or fully reusable rockets are perfected for hypersonic travel, they also lower the bar for entering orbit. We might see synergistic progress – e.g., a hypersonic passenger plane evolving into a spaceplane that can take tourists to orbit (Virgin Galactic’s spaceplane is suborbital and only Mach 3, but future versions could go orbital). By 2050, we could conceive of a single vehicle that can do a London-to-Tokyo hop or go to a space hotel depending on how it’s operated. This kind of dual-use craft is essentially what NASA attempted with the Space Shuttle (which was a hypersonic glider on return, albeit not used for point-to-point travel).
It’s important to temper these possibilities with realism: significant breakthroughs are still required, and delays are almost certain. Aerospace development is costly and often slower than anticipated (the Concorde itself took nearly 20 years from concept to service). Furthermore, the 2030s and 2040s will have to grapple with climate imperatives, which could either spur cleaner high-speed tech or impose constraints that slow adoption (more on this soon).
Nevertheless, as one analyst succinctly put it, “the future of aviation will likely be a mix – subsonic for efficiency, supersonic for urgency, and hypersonic for the extraordinary cases.” Over time, what is extraordinary can become ordinary, as was the case with flight itself or computers or the internet. By the mid-21st century, the vision of routinely zipping around the planet at Mach 5+ might not only be technically feasible but also economically viable and relatively common for a segment of the population. The exact timeline may shift, but the current indicators suggest the future is largely on track for hypersonic flight – barring any massive roadblocks, we are within a couple of decades of seeing the first civilians fly at speeds only experimental craft have achieved to date.
Having painted an optimistic picture of hypersonic possibilities, it’s crucial to address the flipside: the ethical, environmental, and societal implications of this technology. High speeds come with high stakes. As we race toward a hypersonic future, we must consider questions of equitable access, potential misuse, safety, environmental sustainability, and international stability. We turn to these issues next.
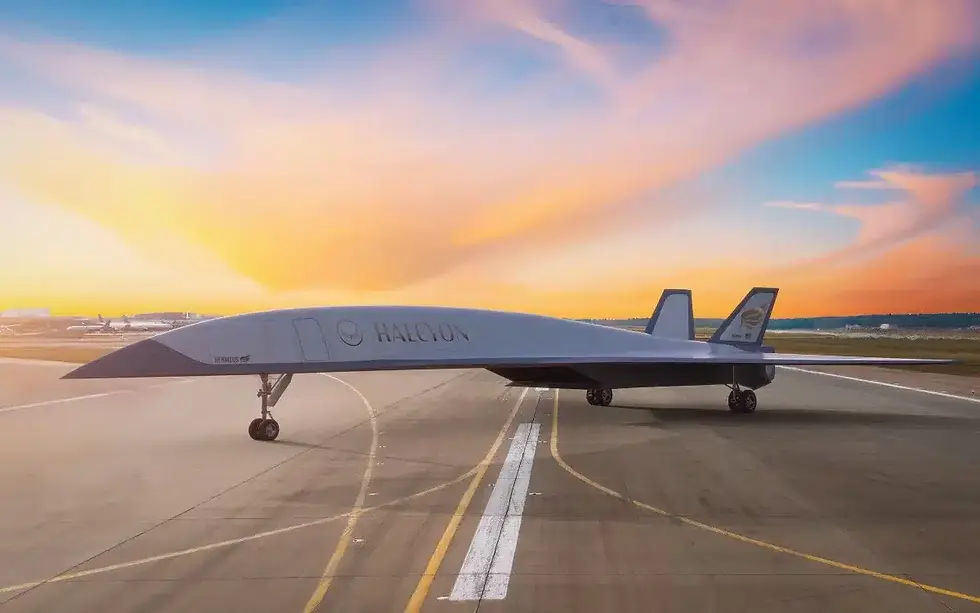
V. Ethical and Societal Implications
The pursuit of hypersonic flight, like many technological advancements, is a double-edged sword. On one edge, it promises progress: faster travel, new industries, strategic deterrence, and scientific discovery. On the other, it raises concerns and challenges that society will have to grapple with. In this section, we explore the broader implications of hypersonic technology, including its dual-use nature (military vs. civilian), issues of accessibility and inequality, privacy and security considerations, environmental impact, and the international geopolitical context. Understanding these aspects is crucial to ensure that the hypersonic age unfolds responsibly and benefits humanity as a whole, not just a select few.
Dual-Use Dilemma and Militarization
Hypersonic technology is inherently dual-use, meaning the same underlying science can serve both military and civilian purposes. This duality presents ethical challenges. On one hand, military investment drives innovation (as we’ve seen, much of the recent progress is thanks to defense-funded programs), which eventually trickles down to civilian benefit. On the other hand, the rush to weaponize hypersonics could lead to a destabilizing arms race and divert resources from peaceful applications.
One concern is that hypersonic weapons – especially those capable of carrying nuclear warheads – may upset the global strategic balance. Because of their speed and maneuverability, they compress decision times for leaders in a crisis and might be seen as “first-strike” weapons. There is a fear that they lower the threshold for miscalculation; a country under a hypersonic attack would have mere minutes (or less) to respond or decide on retaliation. International arms control regimes currently do not specifically cover hypersonic weapons. Unlike ballistic missiles, which have been somewhat regulated by treaties, there is no treaty banning or limiting hypersonic missiles. This absence is partly because the technology is so new – by the time the world witnessed high-profile tests (late 2010s), great-power relations were strained and major arms control agreements were fraying.
The dual-use nature also means that progress in civil hypersonics might be viewed with suspicion. For instance, if a country claims to be testing a hypersonic passenger vehicle, others might worry it’s a cover for weapon development (since a hypersonic airliner could theoretically be adapted to military use, or the research data could apply to missiles). Conversely, a nation might accelerate civil projects precisely to not fall behind technologically if it cannot match others militarily. This dynamic can be seen in how, during the Cold War, the Soviet Union’s early interest in supersonic transports (Tu-144) was partly about not lagging the West technologically and prestige-wise.
From an ethical standpoint, engineers and scientists working on hypersonics face classic dual-use dilemmas: innovations they create for civilian efficiency might be adopted in weapons that can cause destruction. An example is materials engineering – a breakthrough in heat-resistant coatings to enable a jet’s wings to survive Mach 6 could just as easily improve a hypersonic glide weapon’s heat shield, making it more survivable and deadly. Professionals and policymakers will need to navigate these issues, perhaps by establishing norms or agreements. Some experts suggest pursuing arms control for hypersonics, such as mutual transparency measures or even a ban on nuclear-armed hypersonic glide vehicles (to reduce nuclear risk). However, getting major powers to agree may be challenging in the current climate.
On the positive side, military development does not only yield weapons; it can produce valuable civilian spin-offs. The challenge and ethical imperative is to channel as much of the hypersonic R&D as possible into peaceful uses. Governments could, for example, mandate that data from certain declassified tests be shared with civilian agencies like NASA, or provide funding for commercial partnerships (as the USAF is doing with Hermeus).
Accessibility and Inequality
When hypersonic travel becomes available for civilians, who will have access to it? If history is a guide, it will likely be expensive at first – as Concorde was, or as space tourism is today. This raises issues of equity and societal benefit. If hypersonic flight is only accessible to the ultra-rich or to government officials, does it risk becoming a technology that widens the gap between haves and have-nots? For example, a multinational CEO who can afford a hypersonic jet membership might conduct business advantageously around the globe, while competitors who cannot are left at a disadvantage. There is also an intra-national aspect: will only certain countries have hypersonic air travel networks, leaving others behind?
One might argue that this is similar to any new tech (early air travel itself was a luxury for the wealthy). Over time, many technologies do democratize and drop in cost – jet travel is now common, and even supersonic flight might become more affordable if Boom and others succeed with economic models. However, the extreme speeds and costs involved in hypersonic flight may mean it never becomes mass-market in the way subsonic flight did. It could remain a premium service indefinitely, which means its benefits (time saved, etc.) are unevenly distributed.
Societal implications include how we integrate such a tier of travel. Will there be “hypersonic ports” separate from regular airports? Security and safety protocols for these high-speed craft may be different, possibly more stringent. How will air traffic control handle a mix of Mach 5 craft in skies with Mach 0.85 airliners? There’s the possibility of hypersonic corridors or designated altitudes for high-speed travel. These integration issues will require international cooperation and regulatory frameworks – which in turn must be inclusive, so that not only the countries operating hypersonic planes have a say, but also those over whose airspace these craft might fly.
Another ethical facet is ensuring that advances made via public funding (e.g., NASA research) serve broad public interests, not just private companies. If NASA’s 2040 airliner initiative yields technology, one would hope it’s shared to maximize competition and lower costs, rather than locking it up in one corporation’s product that charges exorbitantly.
Privacy and Security
Hypersonic vehicles, especially military ones, introduce some privacy and security concerns. A specific worry is the potential use of high-speed platforms for surveillance. For instance, a hypersonic drone (like a potential SR-72) could overfly any location in a fraction of the time current spy planes or drones take. While satellites already provide a global eye-in-the-sky, a hypersonic surveillance craft could be more responsive (you could dispatch it on-demand to observe somewhere within an hour). This raises questions: will nations feel their airspace is violated if such vehicles routinely pass over (even at high altitude)? There are established norms for satellites (in orbit, above national airspace), but a hypersonic drone flying at 30 km altitude – is that considered sovereign airspace? These legal gray areas need clarity. International law might have to evolve regarding what is permissible at these speeds and altitudes.
From a civilian privacy angle, one could imagine a future with hypersonic transport and perhaps high-altitude hypersonic UAVs for communications or earth observation. While not fundamentally different from existing aircraft, the frequency and speed might concern some (e.g., more sonic booms or contrails overhead). However, privacy is probably less of an issue than security.
Security-wise, consider the risk of hypersonic vehicles being used as weapons in themselves (not just carrying weapons). A hijacked hypersonic airliner could potentially be turned into a kinetic weapon with enormous impact energy (though security for such craft would undoubtedly be very tight). Another angle is cybersecurity: these vehicles will rely on advanced software for stability and navigation at extreme speeds. They could be targets of cyber attacks. A malicious takeover of a hypersonic missile or drone could have catastrophic consequences. Therefore, investing in robust secure control systems is an ethical priority.
For civilian travel, emergency and safety protocols will need a rethink. At Mach 5, if something goes wrong, the crew and passengers have much less time to react or bail out compared to subsonic flight. Ensuring safety to an acceptable level might require innovations like autonomous abort modes or crew escape capsules for hypersonic transports – all of which will influence public acceptance.
Environmental Concerns
Perhaps the most pressing societal issue for hypersonic flight is its environmental impact. We are entering an era where sustainability is paramount; the aviation sector is already under pressure to reduce its carbon footprint. Introducing a class of aircraft that potentially burns significantly more fuel per passenger than conventional jets will face scrutiny. Indeed, research funded by NASA and others has shown that supersonic aircraft designs would likely have much higher fuel burn per passenger-mile – on the order of 5 to 7 times higher than subsonic jets. For hypersonic speeds, the physics implies even greater energy requirements. Pushing a plane to Mach 5+ takes an enormous amount of fuel due to the exponential rise in drag and the energy lost in shock waves and heat.
A study by MIT, for instance, concluded that new supersonic aircraft would consume 3.2 to 10 times more fuel per passenger-mile than subsonic ones. That’s just at Mach 1.6; at Mach 5 the figure could be higher if conventional fuels and engines are used. The ICCT (International Council on Clean Transportation) likewise found high fuel burn for envisioned supersonic business jets, reinforcing that these planes would be “major polluters” if flown with current fuel technology. As one expert starkly put it: “There is no middle space where supersonic aircraft succeed and they aren’t a major polluter – that future basically doesn’t exist.” This admonition suggests that unless the sector finds solutions like sustainable aviation fuels (SAF), hydrogen fuel, or electric/hybrid propulsion (which is far off for hypersonics), the climate impact could be severe.
Beyond CO₂ emissions, high-altitude emissions have disproportionate effects. Supersonic and hypersonic jets would fly in the stratosphere (15–30 km altitude). Exhaust from hydrocarbon fuels at that height can affect the ozone layer and produce persistent greenhouse effects. Water vapor from rockets, for example, can accumulate in the stratosphere and contribute to ozone depletion or warming. Additionally, sonic booms are a form of noise pollution. Hypersonic vehicles, especially during acceleration and reentry phases, will create sonic booms that could disturb communities if flights are over land. Current regulations ban civil supersonic over land specifically because of sonic boom annoyance. Hypersonic booms would likely be even more intense (higher speed = stronger shock, though higher altitude might dissipate some). NASA’s work on low-boom shaping might help, but doing that at Mach 5 is a new challenge.
Another environmental aspect is the manufacturing and materials. Hypersonic vehicles might use exotic materials (titanium, carbon composites, etc.) whose production is energy-intensive and polluting. For instance, carbon fiber manufacturing has a high carbon footprint. If a fleet of hypersonic jets requires a lot of rare materials or energy to produce, that adds to their environmental cost.
Given these concerns, there is a real possibility that environmental regulations could slow or limit hypersonic aviation development. Future carbon pricing or emissions trading schemes might make operating a Mach 5 airliner prohibitively expensive unless it’s carbon-neutral. We are already seeing pushback on even the idea of supersonic travel: environmental groups argue that it’s a step backward in sustainability. To address this, companies are researching alternative fuels. One could imagine a hypersonic plane powered by “green hydrogen” (hydrogen produced from renewable energy) – that would emit mainly water vapor, no CO₂, though water at high altitude is also a greenhouse gas to consider. Another idea is synthetic fuels or biofuels that are net-zero carbon. However, those alone don’t solve the NOx emissions and other impacts at high altitude.
Thus, an ethical imperative for engineers is to integrate climate considerations from day one in hypersonic designs. Perhaps that means trading a bit of speed or range for a lot more efficiency. For example, instead of Mach 5, maybe a Mach 4 aircraft with hybrid propulsion could drastically cut fuel burn. Or designing vehicles to fly lower (in denser air) during cruise could reduce NOx formation (though it increases drag). Some have suggested optimizing routes to avoid sensitive ozone regions of the atmosphere.
In summary on environment: Without mitigation, hypersonic flight could significantly worsen aviation’s environmental footprint at a time when it needs to shrink. Balancing the desire for speed with the necessity of sustainability is one of the biggest societal challenges for this field. If the industry fails to do so, it may face public opposition or regulatory bans (for instance, if international bodies conclude the climate impact is unacceptable). On the other hand, solving these problems (like developing a carbon-neutral hypersonic propulsion) would be a massive win that could justify the technology’s broad adoption.
International Cooperation vs. Competition
Hypersonic flight lies at the intersection of international competition and cooperation. As we’ve covered, there’s an ongoing race among great powers to develop hypersonic weapons. This competitive aspect can breed mistrust and reduce information sharing; for example, China and Russia have revealed some capabilities but keep details secret, while the U.S. programs are often classified. This secrecy can slow overall progress (since researchers cannot openly collaborate) and increase the risk of misunderstandings.
However, there are also avenues for international cooperation:
In the civilian realm, joint projects could share the burden of R&D. For instance, a hypothetical future “Hyperliner” project might be an international consortium (like Airbus, but even broader) pooling expertise from multiple countries. Europe has had some early concepts (the LAPCAT project, for example, studied a Mach 8 hydrogen-powered plane with contributions from various EU countries).
Standards and regulations for hypersonic operations would benefit from global coordination. Organizations like ICAO (International Civil Aviation Organization) and IATA will likely get involved to set guidelines on things like permissible sonic boom levels, recommended practices for hypersonic flight corridors, etc., just as they do for supersonic.
Even militarily, there could be dialogues or confidence-building measures. Some analysts propose creating data exchange agreements or notifications for hypersonic missile tests (similar to what exists for ICBM launches) to reduce the chance of a test being mistaken for an attack. Another idea is a hypersonic arms control agreement where nations might agree not to deploy certain types or ranges of hypersonic missiles, in return for others doing the same. It’s admittedly difficult in the current geopolitical climate, but not impossible if there’s recognition that an uncontrolled arms race serves no one’s interest.
We should note an interesting nuance: dual-use technology flow between allies. The U.S., for example, is working with Australia and the U.K. (under AUKUS pact) on hypersonic research and defense. This means allied countries pooling resources to compete with adversaries. While cooperative within the bloc, it’s still competitive globally.
Finally, there’s the perspective of countries that aren’t directly developing hypersonics. They might worry about being left vulnerable or left out of a new transportation revolution. If global regimes like the United Nations could get involved, they might push for hypersonic tech to be shared for peaceful uses under some frameworks (similar to how nuclear technology is shared for civilian power under the IAEA’s watch, despite military nuclear proliferation being controlled).
In a positive scenario, hypersonic flight could become a field where scientists collaborate internationally – say, a global hypersonic research center or an extension of something like CERN into aerodynamics. This could speed up breakthroughs and ensure all humanity benefits. The European Space Agency (ESA), NASA, JAXA (Japan), and others have occasionally collaborated on high-speed research (ESA’s HEXAFLY-INT project involved partners from Europe, Russia, Australia, and others to test a hypersonic glider concept). Such collaborations build trust and share knowledge.
In conclusion of cooperation vs. competition: The path we take will influence whether hypersonic flight becomes a unifying achievement or a source of tension. Ethically, many argue for transparency and engagement: if nations are open about their testing and willing to discuss rules of the road, the technology can progress with reduced risk of conflict. The societal benefit of hypersonic commercial travel also depends on peace – obviously, if these technologies spark conflict, civilian uses will suffer. So, working towards some level of international understanding regarding hypersonics is an important, if challenging, task ahead.
Balancing Progress and Responsibility
The above topics illustrate that the hypersonic revolution must be guided responsibly. We must weigh the thrill of speed with wisdom. Some key balanced approaches include:
Phased Introduction: Instead of unleashing hypersonic travel everywhere at once, start with pilot programs, specific routes, and thoroughly study their impacts (noise, environment, safety) before scaling up.
Regulation and Oversight: Governments and international bodies should proactively set standards for hypersonic vehicles. This could include requiring the use of greener fuels or imposing route restrictions to avoid populated areas with sonic booms. Having these rules in place early will signal to innovators that solutions must be found, rather than trying to retrofit fixes after issues arise.
Public Engagement: Supersonic and hypersonic projects should engage with communities and stakeholders. Concorde faced public opposition in its day (over sonic booms and ozone concerns); future projects would do well to involve the public in discussions – for example, sharing sonic boom mitigation plans, or getting input on acceptable noise levels. Engaging environmental groups, as adversarial as it might seem, could also lead to better designs or compromises.
Ensuring Ethical Use: For military use, leaders could commit to principles like not arming hypersonic weapons with nuclear warheads (some U.S. officials have already indicated they plan only conventional payloads, which reduces the doomsday risk somewhat). They could also pursue missile defense research for hypersonics, to provide a sense of security that might make nations feel less pressured to have a hair-trigger response. This is tricky – defenses are lagging behind offense in this case – but it’s part of the equation.
Education and Workforce: A societal implication is preparing people to work in this high-tech field. Governments and industries should train the next generation of engineers in hypersonic science. This will ensure we have the human capital to innovate safely and efficiently. It also democratizes the field so it’s not just a small clique of specialists.
Contingency Planning: Hypersonic vehicles will pose new scenarios for emergency response. What if one crashes in a populated area, scattering exotic materials or causing a fire at extreme temperature? Authorities will need plans for such scenarios (special firefighting techniques, evacuation plans for a rocket launch failure, etc.). Being prepared will mitigate potential disasters.
As we stand on the cusp of this new era, society has the chance to steer hypersonic technology toward net positive outcomes. Just as the jet age brought people together and enabled globalization (despite being born from military jets of WWII), the hypersonic age can bring its share of good – but likely only if we consciously address its downsides from the start.
VI. Final Thoughts
Hypersonic flight represents one of the most exciting frontiers in aerospace today. The phrase “Is the future already here?” captures the essence of this moment: after decades of research and sporadic breakthroughs, we are finally witnessing the translation of hypersonic theory into tangible reality. In military laboratories and in startup garages, vehicles that streak through the sky at five to ten times the speed of sound are being built and tested. The physics behind hypersonic flight, once an esoteric subject, is now the foundation for practical engineering solutions to real-world goals – whether that’s deterring adversaries or shrinking travel times for global citizens.
This deep-dive has explored how we got here (from the X-15 and Concorde to today’s X-51, HAWC, and beyond), what is happening now (a flurry of projects from scramjet missiles to Mach 5 airliner concepts), what might come next (forecasts of supersonic business flights in the 2030s and perhaps civilian hypersonic craft by the 2040s), and what challenges we must confront (ethical, environmental, and geopolitical). The picture that emerges is nuanced. Yes, the future is arriving faster than ever – literally – but it comes with strings attached.
One striking realization is that hypersonic flight is not entirely unprecedented; every crewed spacecraft reentry has been a hypersonic journey, and experimental pilots tasted this regime decades ago. What’s new is the ambition to sustain and utilize hypersonic flight within the atmosphere for useful missions. We’re moving from one-off stunts to potentially routine operations. In that sense, the future isn’t suddenly here; it’s been creeping up on us, and we are at the tipping point where it transitions from experiment to experience.
For the tech-savvy and affluent reader, one might ask: When can I fly hypersonic? If you’re in the military or a government role, perhaps within the next decade you might hitch a ride on a hypersonic test craft or see a drone in action. For commercial passengers, realistic estimates suggest it’s a couple of decades out for any kind of broader service. But there is a high probability that in our lifetime, we will see passengers boarding a vehicle – be it a specialized airplane or a rocket – and landing halfway across the world in the time it takes to watch a movie.
Consider the symbolic significance: the first era of aviation was about connecting cities and continents (the 20th century saw air travel go from nothing to an everyday miracle). The second era might be about erasing the barrier of time – making distance almost irrelevant. Hypersonic and suborbital flights could compress the world into a truly global community. That can foster greater understanding and economic growth, much as jets did, but multiplied. Of course, that’s the optimistic outcome. We have discussed the perils – an arms race, climate damage, exclusivity – which are not inevitable if we manage this technology wisely.
In pondering “is the future already here,” we can say pieces of it are: A rocket glider did fly at Mach 9.6; a warhead is gliding in Earth’s atmosphere at Mach 20 in Russian silos; a startup engine fired that can transition to ramjet mode in a blink. These achievements are to the 2020s what the sound barrier break was to the 1940s – proof points that will likely be seen in hindsight as the start of a new chapter. The full narrative of that chapter – widespread hypersonic flight – is still being written, and we are all stakeholders in how it unfolds.
To conclude, the future of hypersonic flight stands at a thrilling intersection of science, engineering, policy, and imagination. The physics behind it – shock waves, plasma sheaths, temperatures that turn air to fire – is as extreme as physics gets in our atmosphere. Mastering those physics is a testament to human ingenuity. Now, applying that mastery wisely is the next test.
We should celebrate the breakthroughs: the awe of an object piercing through the sky faster than a rifle bullet, the brilliance of an engine that can breathe air at unimaginable speed, the courage of those pushing the envelope. At the same time, we should remain critical and conscientious about why we pursue this speed and how we implement it. The question “Is the future already here?” invites us not just to marvel at the technology but also to reflect on our readiness – technologically, socially, and ethically – for that future.
In the end, hypersonic flight, like the jet engine and the rocket before it, is a tool. It’s up to us to shape it into an instrument of progress. If we do, then the hypersonic age will indeed be a leap forward that future generations may look back on with gratitude, much as we look back on the dawn of flight. The horizon is beckoning at Mach 5 – let’s ensure we cross it responsibly and reap the rewards for all of humankind.

VII. References
Definition of Hypersonic Flight: Wikipedia – “Hypersonic flight is flight through the atmosphere below altitudes of about 90 km at speeds greater than Mach 5, a speed where dissociation of air begins to become significant and high heat loads exist.” This provides the technical threshold and conditions that define the hypersonic regime, emphasizing the onset of extreme aerodynamic heating and chemical effects in air.
X-15 Historical Speed Record: NASA Dryden / Wikipedia – The X-15 rocket plane, flown by USAF Major Pete Knight in 1967, reached approximately Mach 6.7 (around 7,200 km/h), which remains one of the fastest speeds recorded by a crewed aircraft in atmosphere. This is a key historical milestone demonstrating early human entry into hypersonic speeds.
NASA X-43A and X-51 Scramjet Achievements: Aerospace Tech / Wikipedia – In 2004, NASA’s unmanned X-43A scramjet demonstrator flew at about Mach 9.6 for a brief period, and in 2013 the Boeing X-51 Waverider sustained Mach 5.1 for over 200 seconds of scramjet-powered flight. These successes proved the viability of air-breathing propulsion in the hypersonic regime.
HAWC Program Success: DARPA Release / Space.com – In January 2023, DARPA’s HAWC project had its final test where a scramjet-powered vehicle, released from a B-52, flew over 300 nautical miles and above 60,000 ft at speeds greater than Mach 5, meeting all objectives. This marked a significant step in developing a hypersonic cruise missile capability.
Russian Hypersonic Systems (Kinzhal, Avangard, Zircon): VOA News – Russia has deployed the Kinzhal, an air-launched ballistic missile reaching up to Mach 10 with 2,000 km range, as well as the Avangard HGV, claimed to exceed Mach 20 (over 10,000 km range) and the ship-launched Zircon cruise missile (Mach 8 top speed, 1,000 km range). These figures underscore the advanced state of Russian hypersonic weaponry as of mid-2020s.
Chinese Hypersonic Systems (DF-17 and Orbital Test): VOA News – China’s DF-17 medium-range missile uses a hypersonic glide vehicle and is operational (~1,600–2,000 km range). Notably, in a July 2021 test, China launched a hypersonic glide vehicle that circled the globe in low orbit before reentering, demonstrating a fractional orbital bombardment capability that surprised U.S. officials. This highlights China’s leading-edge experiments in long-range hypersonics.
Boeing Hypersonic Airliner Concept and Timeline: Aircraft Interiors International – Boeing revealed a vision for a Mach 5 passenger aircraft that could be flying in 2040, featuring a sharp-nosed, twin-tail design capable of transatlantic flights in ~2 hours. This indicates industry expectations for when a civilian hypersonic airliner might become feasible and the performance it would offer.
NASA Contracts for High-Speed Commercial Flight: Military Aerospace News – In May 2023, NASA awarded Boeing and Northrop Grumman $5.1 million to study “High-Speed Endoatmospheric Commercial Vehicles” targeting Mach 2–5 speeds, with the aim of an environmentally sustainable high-speed passenger transport entering service in the 2030s. This shows official support for and belief in reviving supersonic/hypersonic travel in the civilian sector within the next decade or two.
Environmental Impact of Supersonic/Hypersonic Aircraft: Inside Climate News / ICCT analysis – Research indicates future supersonic aircraft would burn 3 to 10 times more fuel per passenger-mile than subsonic jets, which would greatly increase emissions. Experts warn “there is no scenario” in which they are not major polluters without mitigation. This underscores the environmental challenges high-speed air travel faces, emphasizing the need for sustainable solutions as part of development.
Quote on Dual-Use and Arms Race Concerns: War on the Rocks / Arms Control Association – “China, Russia, and the United States are all developing hypersonic missiles, albeit with mixed success…hypersonic missiles are highly maneuverable despite flying at Mach 5…making them especially difficult to detect and stop”. This analysis points out both the widespread pursuit of hypersonic arms and the threat they pose to current defense systems, fueling an arms race dynamic.
Comments